Where Do Chemosynthetic Get Their Energy
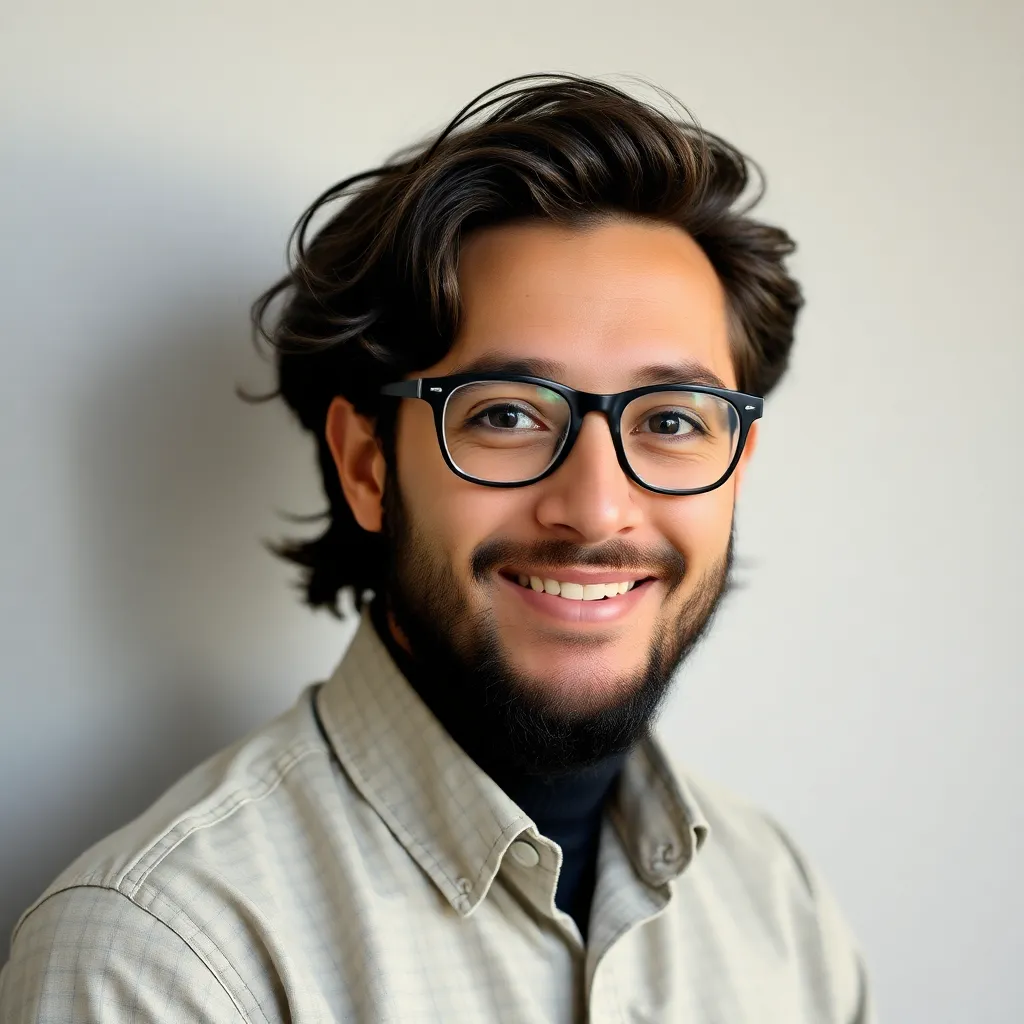
Muz Play
Apr 22, 2025 · 6 min read

Table of Contents
Where Do Chemosynthetic Organisms Get Their Energy?
Chemosynthesis, a fascinating process in the biological world, provides a crucial alternative to photosynthesis as a means of energy production. Unlike photosynthetic organisms that rely on sunlight, chemosynthetic organisms, also known as chemoautotrophs, obtain energy from the oxidation of inorganic compounds. This process supports a wide array of life forms in diverse, often extreme, environments across the globe. Understanding where these organisms get their energy requires delving into the specifics of their metabolic pathways and the unique environments they inhabit.
The Fundamentals of Chemosynthesis
Chemosynthesis is fundamentally a process of chemical energy conversion. Instead of harnessing light energy like plants and algae, chemosynthetic organisms utilize the energy released from chemical reactions involving inorganic molecules. This energy is then used to drive the synthesis of organic compounds, primarily carbohydrates, which serve as the building blocks for cellular growth and reproduction. The core process involves the transfer of electrons from an electron donor (the inorganic compound) to an electron acceptor (often oxygen), releasing energy in the form of ATP (adenosine triphosphate), the universal energy currency of cells.
Key Differences from Photosynthesis
While both chemosynthesis and photosynthesis generate organic matter, they differ significantly in their energy source and the electron donors involved:
- Energy Source: Photosynthesis uses light energy, whereas chemosynthesis uses chemical energy.
- Electron Donor: Photosynthesis uses water as the primary electron donor, releasing oxygen as a byproduct. Chemosynthesis utilizes a variety of inorganic electron donors, such as hydrogen sulfide, methane, ammonia, and ferrous iron, resulting in diverse byproducts.
This fundamental difference in energy acquisition allows chemosynthetic organisms to thrive in environments devoid of sunlight, such as deep-sea hydrothermal vents, caves, and soil layers.
Diverse Energy Sources for Chemosynthesis
The remarkable adaptability of chemosynthetic organisms is reflected in the wide range of inorganic compounds they can utilize as energy sources. These diverse sources determine the specific types of chemosynthetic pathways employed and the unique environments these organisms inhabit.
1. Hydrogen Sulfide (H₂S) Oxidation: The Deep-Sea Vent Ecosystem
Arguably the most well-known example of chemosynthesis involves the oxidation of hydrogen sulfide (H₂S). This process is pivotal to the remarkable ecosystems surrounding deep-sea hydrothermal vents. These vents release superheated, mineral-rich water, which is rich in H₂S. Bacteria and archaea inhabiting these vents utilize H₂S as an electron donor, oxidizing it to elemental sulfur or sulfate (SO₄²⁻). The energy released from this oxidation is used to fix carbon dioxide (CO₂) into organic molecules, forming the base of the food web for a variety of unique vent organisms, including tubeworms, mussels, and clams.
The process can be summarized as follows:
H₂S + O₂ → S⁰ + H₂O + Energy
This energy is then used to drive the fixation of CO₂, ultimately leading to the synthesis of organic compounds.
2. Methane (CH₄) Oxidation: A Versatile Energy Source
Methane, a potent greenhouse gas, is another significant energy source for chemosynthetic organisms. Methanotrophic bacteria and archaea are capable of oxidizing methane, using the released energy to produce organic matter. These organisms are found in various anaerobic environments, including deep-sea sediments, rice paddies, and even some soils. Methane oxidation plays a critical role in the global carbon cycle, removing substantial amounts of methane from the atmosphere.
The basic reaction is:
CH₄ + O₂ → CO₂ + H₂O + Energy
3. Ammonia (NH₃) Oxidation: The Nitrogen Cycle Connection
Ammonia oxidation is a crucial process in the nitrogen cycle, driven by ammonia-oxidizing archaea and bacteria. These organisms oxidize ammonia (NH₃) to nitrite (NO₂⁻), releasing energy in the process. Nitrite is further oxidized to nitrate (NO₃⁻) by other bacteria, completing the nitrification process. This process is essential for nutrient cycling in various environments, including soils, oceans, and wastewater treatment plants.
The reaction steps are:
NH₃ + O₂ → NO₂⁻ + H⁺ + H₂O + Energy NO₂⁻ + O₂ → NO₃⁻ + Energy
4. Iron (Fe²⁺) Oxidation: Ancient and Widespread
Iron oxidation is one of the oldest forms of chemosynthesis, dating back to early Earth. Iron-oxidizing bacteria utilize ferrous iron (Fe²⁺) as an electron donor, oxidizing it to ferric iron (Fe³⁺). This process plays a significant role in iron cycling in various environments, including acidic mine drainage, soils, and marine sediments. While the energy yield from iron oxidation is relatively low compared to other chemosynthetic processes, these organisms contribute significantly to iron transformations in the environment.
The basic reaction is:
Fe²⁺ + 1/4 O₂ + H⁺ → Fe³⁺ + 1/2 H₂O + Energy
Chemosynthesis: A Critical Role in Global Ecosystems
Chemosynthesis is not just a niche process confined to extreme environments. It plays a surprisingly significant role in global biogeochemical cycles and supports substantial biodiversity.
1. Supporting Deep-Sea Ecosystems
Deep-sea hydrothermal vents, fueled by chemosynthesis, represent some of the most extraordinary ecosystems on Earth. These environments, devoid of sunlight, support unique communities of organisms completely independent of photosynthesis. The chemosynthetic bacteria form the base of the food web, providing sustenance for a variety of invertebrates and even some fish species. The discovery of these vent ecosystems greatly expanded our understanding of life's adaptability and the potential for life to exist in seemingly inhospitable environments.
2. Influence on Global Carbon and Nutrient Cycling
Chemosynthetic processes significantly impact global biogeochemical cycles. Methane oxidation, for instance, plays a major role in regulating atmospheric methane concentrations, mitigating its impact on climate change. Ammonia oxidation is essential for nitrogen cycling, providing a crucial link between inorganic and organic nitrogen compounds. Iron oxidation, although less energy-yielding, plays a crucial role in iron cycling, influencing ocean acidification and nutrient availability.
3. Potential for Astrobiological Implications
The existence of chemosynthetic life in extreme environments on Earth raises intriguing possibilities for extraterrestrial life. If life can thrive on chemical energy in the absence of sunlight, the potential for life on other planets, particularly those with subsurface oceans or hydrothermal activity, becomes significantly more plausible. The study of chemosynthetic organisms provides crucial insights into the potential for life beyond Earth and expands our understanding of the limits of habitability.
Conclusion
Chemosynthesis, a process of energy production based on the oxidation of inorganic compounds, supports a surprisingly diverse range of life forms across various environments. From the depths of the ocean to the soil beneath our feet, these organisms utilize a wide variety of chemical energy sources, playing crucial roles in global biogeochemical cycles and demonstrating the remarkable adaptability of life. Further research into chemosynthetic processes will undoubtedly reveal more about the intricate workings of these unique ecosystems and their implications for understanding life on Earth and beyond. The study of chemosynthesis not only enhances our biological knowledge but also informs our understanding of potential life on other planets and the resilience of life in extreme conditions. Understanding how these organisms harness chemical energy opens up exciting possibilities for future research and exploration.
Latest Posts
Latest Posts
-
Which Change Of State Involves A Release Of Energy
Apr 22, 2025
-
How To Write A System Of Linear Equations
Apr 22, 2025
-
What Type Of Rock Contains Large Crystals
Apr 22, 2025
-
What Is A Membrane Bound Organelles
Apr 22, 2025
-
What Element Has 5 Protons And 6 Neutrons
Apr 22, 2025
Related Post
Thank you for visiting our website which covers about Where Do Chemosynthetic Get Their Energy . We hope the information provided has been useful to you. Feel free to contact us if you have any questions or need further assistance. See you next time and don't miss to bookmark.