Where Do Plants Get The Energy To Make Organic Molecules
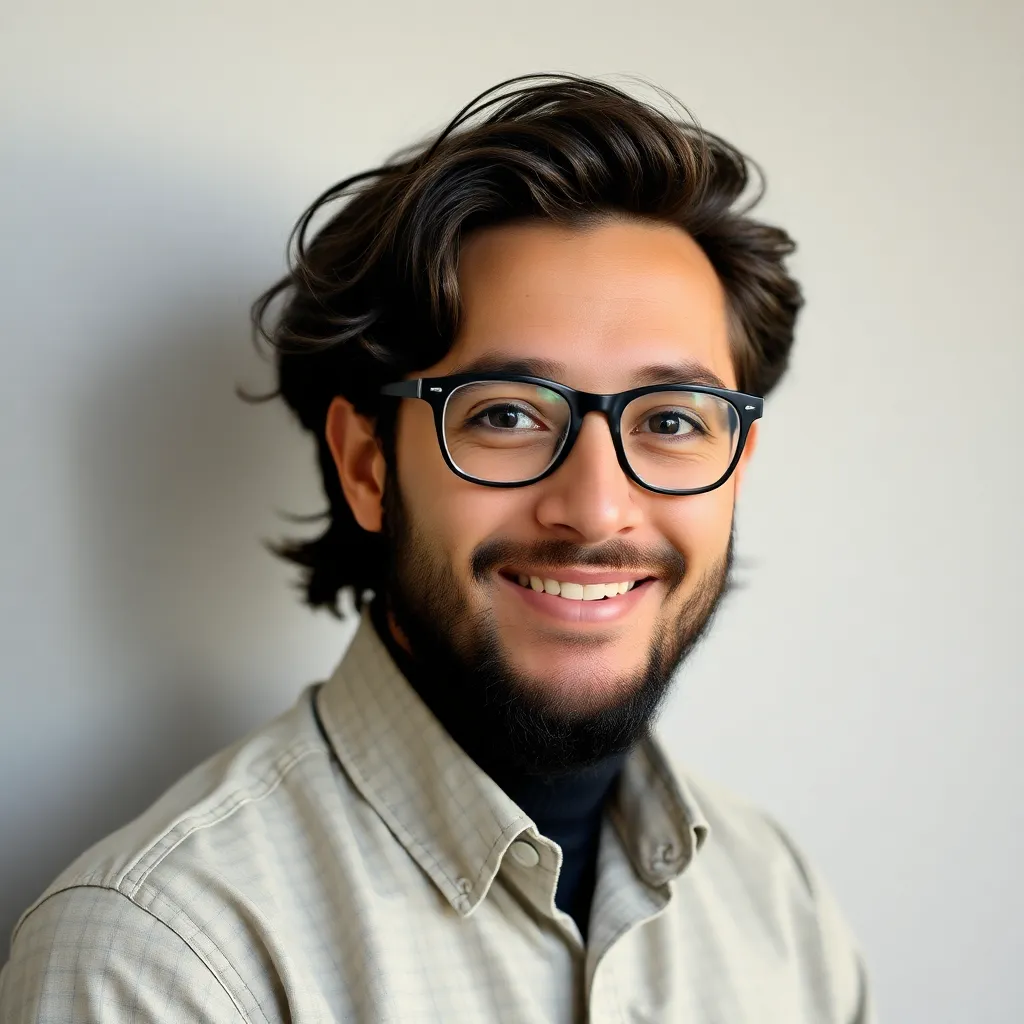
Muz Play
Apr 21, 2025 · 6 min read

Table of Contents
Where Do Plants Get the Energy to Make Organic Molecules?
Plants, the silent architects of our planet's ecosystems, are masters of bio-chemical engineering. They create their own food, a process known as photosynthesis, which is fundamental to life on Earth. But where do they obtain the energy to fuel this incredible feat of organic molecule synthesis? The answer lies in the sun, the ultimate power source for almost all life.
The Sun: The Primary Energy Source
The sun is a colossal nuclear furnace, constantly emitting radiant energy in the form of light and heat. This energy, primarily in the visible light spectrum, is captured by plants and converted into chemical energy, stored within the bonds of organic molecules like glucose. This energy conversion process, photosynthesis, is at the heart of understanding plant life.
Electromagnetic Radiation and Photosynthetic Pigments
Sunlight is composed of various wavelengths of electromagnetic radiation. Plants, however, don't utilize all of these wavelengths equally. They've evolved specialized molecules, called photosynthetic pigments, to absorb specific wavelengths effectively. The most important of these is chlorophyll, which absorbs strongly in the blue and red regions of the visible spectrum, reflecting green light (hence the green color of most plants).
Chlorophyll a and chlorophyll b are the primary chlorophyll types involved in photosynthesis. These pigments are embedded within complex protein structures called photosystems, located within the thylakoid membranes of chloroplasts—the plant cell's specialized organelles for photosynthesis. Accessory pigments like carotenoids and phycobilins also play vital roles, absorbing light energy that chlorophyll misses and transferring this energy to chlorophyll for use in photosynthesis. This broad absorption spectrum ensures that plants can maximize their energy intake from sunlight, even under varying light conditions.
Light Absorption and Excitation of Electrons
When a photon (a particle of light) strikes a chlorophyll molecule, its energy is absorbed. This energy boosts an electron within the chlorophyll molecule to a higher energy level, a process called excitation. This excited electron is now highly reactive and unstable, and its energy is harnessed to drive the biochemical reactions of photosynthesis.
The Two Stages of Photosynthesis: Light-Dependent and Light-Independent Reactions
Photosynthesis is a two-stage process:
-
Light-dependent reactions: These reactions occur in the thylakoid membranes of the chloroplasts and directly utilize light energy to produce ATP (adenosine triphosphate) and NADPH (nicotinamide adenine dinucleotide phosphate). ATP and NADPH are energy-carrying molecules that act as the power source for the next stage. This stage involves two photosystems, Photosystem II (PSII) and Photosystem I (PSI), working in tandem.
-
Light-independent reactions (Calvin Cycle): These reactions, also known as the dark reactions (despite not occurring solely in the dark), take place in the stroma of the chloroplasts. They utilize the ATP and NADPH generated in the light-dependent reactions to convert carbon dioxide (CO2) from the atmosphere into glucose and other organic molecules. This process is a crucial example of carbon fixation, converting inorganic carbon into organic forms usable by the plant.
Detailed Breakdown of Light-Dependent Reactions
The light-dependent reactions are a series of intricate electron transfer events. Here's a simplified overview:
-
Photosystem II (PSII): Light energy excites electrons in chlorophyll molecules within PSII. These high-energy electrons are passed along an electron transport chain, a series of protein complexes embedded in the thylakoid membrane. As electrons move down the chain, energy is released, used to pump protons (H+) from the stroma into the thylakoid lumen, creating a proton gradient. This gradient drives the synthesis of ATP via chemiosmosis, a process where protons flow back into the stroma through ATP synthase, an enzyme that catalyzes ATP production. The electrons lost by PSII are replaced by electrons derived from the splitting of water molecules (photolysis), releasing oxygen as a byproduct—the oxygen we breathe.
-
Photosystem I (PSI): Electrons from PSII reach PSI, where they are again excited by light energy. These energized electrons are then passed to another electron transport chain, ultimately reducing NADP+ to NADPH. NADPH serves as a reducing agent, carrying high-energy electrons that will be used in the Calvin Cycle to reduce carbon dioxide.
Detailed Breakdown of Light-Independent Reactions (Calvin Cycle)
The Calvin Cycle is a cyclical series of reactions that fixes atmospheric CO2 into organic molecules. This process can be summarized in three main stages:
-
Carbon Fixation: CO2 combines with a five-carbon molecule called RuBP (ribulose-1,5-bisphosphate), catalyzed by the enzyme RuBisCO (ribulose-1,5-bisphosphate carboxylase/oxygenase). This reaction produces an unstable six-carbon intermediate that quickly breaks down into two molecules of 3-PGA (3-phosphoglycerate).
-
Reduction: ATP and NADPH from the light-dependent reactions provide the energy and reducing power to convert 3-PGA into G3P (glyceraldehyde-3-phosphate), a three-carbon sugar.
-
Regeneration: Some G3P molecules are used to synthesize glucose and other organic molecules, while the rest are recycled to regenerate RuBP, ensuring the cycle's continuation.
Beyond Glucose: A Diverse Range of Organic Molecules
While glucose is a primary product of photosynthesis, plants utilize the energy stored within glucose to synthesize a vast array of other organic molecules, including:
- Starch: A storage polysaccharide, providing a long-term energy reserve.
- Cellulose: A structural polysaccharide, forming the cell walls of plants.
- Proteins: Essential for building and maintaining plant tissues, composed of amino acids. Plants synthesize amino acids using the carbon skeletons derived from the Calvin Cycle and nitrogen obtained from the soil.
- Lipids (fats and oils): Important for energy storage, membrane structure, and hormone production.
- Nucleic Acids (DNA and RNA): Essential for genetic information storage and protein synthesis.
The synthesis of these diverse molecules requires energy derived initially from sunlight, captured through photosynthesis and stored in the chemical bonds of organic molecules.
Factors Affecting Photosynthesis
Several factors influence the rate of photosynthesis:
-
Light Intensity: Increasing light intensity generally increases photosynthesis until a saturation point is reached, where further increases have little effect.
-
Carbon Dioxide Concentration: Higher CO2 levels generally increase photosynthesis until a saturation point is reached.
-
Temperature: Photosynthesis is temperature-dependent, with optimal temperatures varying depending on the plant species. High temperatures can denature enzymes involved in photosynthesis, reducing efficiency.
-
Water Availability: Water is essential for photosynthesis, and water stress can significantly reduce photosynthetic rates.
The Importance of Photosynthesis for Life on Earth
Photosynthesis is the cornerstone of most food webs on Earth. It provides the primary source of energy and organic carbon for nearly all living organisms, either directly (through plant consumption) or indirectly (through consumption of herbivores, carnivores, etc.). Without photosynthesis, the planet's ecosystems would collapse, and life as we know it would be impossible.
The oxygen produced as a byproduct of photosynthesis is also crucial for the survival of aerobic organisms, including humans. This oxygen is essential for cellular respiration, the process by which we convert the energy stored in organic molecules into ATP to power our cells.
Conclusion: A Continuous Cycle of Energy Transformation
Plants harness the energy from the sun, converting it into chemical energy stored within organic molecules. This process, photosynthesis, is a remarkable feat of biological engineering, driving the growth and survival of plants and underpinning the entire biosphere. Understanding the intricate mechanisms of photosynthesis is crucial for comprehending the complexities of life on Earth and for developing sustainable solutions to global challenges, such as climate change and food security. By optimizing photosynthetic efficiency, we can potentially enhance crop yields and contribute to a more sustainable future. The sun's energy, captured and transformed by plants, fuels the very existence of our planet's vibrant and diverse ecosystems.
Latest Posts
Latest Posts
-
Which Units Express Specific Heat Capacity
Apr 21, 2025
-
Calculate The Moles Of H2so4 In Titration
Apr 21, 2025
-
Which Group Tends Not To Form Ions Or React
Apr 21, 2025
-
In The Figure Where Is Atp Produced
Apr 21, 2025
-
In The Citric Acid Cycle Atp Molecules Are Produced By
Apr 21, 2025
Related Post
Thank you for visiting our website which covers about Where Do Plants Get The Energy To Make Organic Molecules . We hope the information provided has been useful to you. Feel free to contact us if you have any questions or need further assistance. See you next time and don't miss to bookmark.