Where Does Cellular Respiration Take Place In Eukaryotes
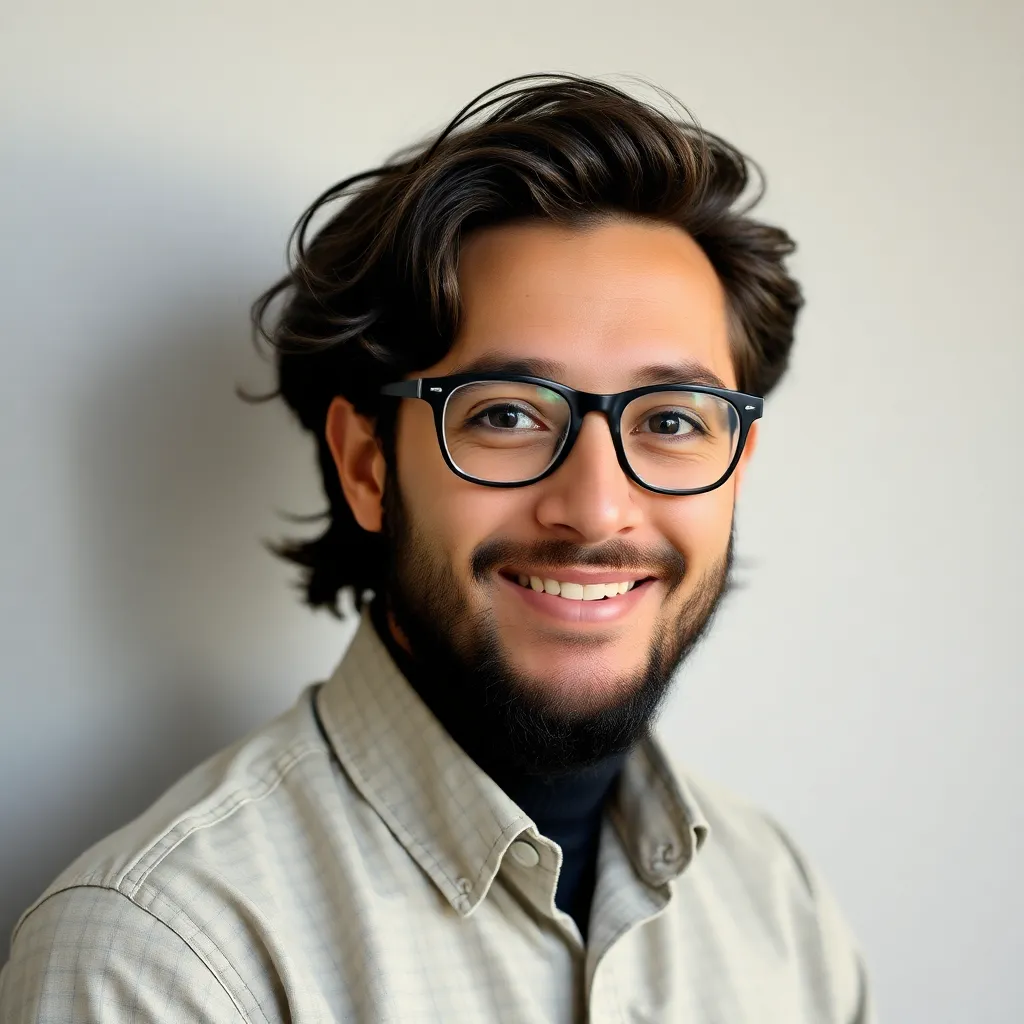
Muz Play
Apr 14, 2025 · 6 min read

Table of Contents
Where Does Cellular Respiration Take Place in Eukaryotes? A Deep Dive into the Cellular Powerhouse
Cellular respiration, the process by which cells break down glucose to generate energy in the form of ATP (adenosine triphosphate), is fundamental to life. Understanding where this intricate process unfolds within eukaryotic cells is crucial to grasping the complexity and efficiency of biological energy production. While prokaryotes carry out respiration in their cytoplasm, the compartmentalization of eukaryotic cells allows for a highly organized and optimized system. This article delves into the specific locations within eukaryotic cells where each stage of cellular respiration occurs, exploring the roles of various organelles and the intricate interplay between them.
The Main Players: Mitochondria – The Powerhouses of the Cell
The most significant player in eukaryotic cellular respiration is undoubtedly the mitochondrion. These double-membraned organelles, often referred to as the "powerhouses of the cell," are the primary sites for the majority of ATP production. Their unique structure, with an outer and inner membrane separated by the intermembrane space, is crucial for the efficient operation of the electron transport chain and oxidative phosphorylation, the final stages of cellular respiration.
The Outer Mitochondrial Membrane: A Selectively Permeable Barrier
The outer mitochondrial membrane is relatively permeable due to the presence of porins, large channel proteins that allow the passage of small molecules and ions. This permeability ensures that the necessary substrates for respiration can readily access the inner mitochondrial compartments.
The Intermembrane Space: A Crucial Electrochemical Gradient
The space between the outer and inner mitochondrial membranes, the intermembrane space, plays a vital role in establishing a proton gradient. This gradient, a difference in proton (H⁺) concentration and electrical potential across the inner mitochondrial membrane, is the driving force behind ATP synthesis. The accumulation of protons in the intermembrane space during the electron transport chain creates the electrochemical potential energy necessary for ATP production.
The Inner Mitochondrial Membrane: The Site of Oxidative Phosphorylation
The inner mitochondrial membrane is highly folded into structures called cristae. This extensive folding dramatically increases the surface area available for the enzymes and protein complexes involved in the electron transport chain and ATP synthase. The inner membrane is impermeable to most ions and molecules, maintaining the integrity of the proton gradient. This impermeability is key to the efficiency of ATP synthesis via chemiosmosis. Electron transport chain complexes (I-IV) and ATP synthase are embedded within the inner mitochondrial membrane.
The Mitochondrial Matrix: The Site of Pyruvate Oxidation and the Krebs Cycle
The mitochondrial matrix, the space enclosed by the inner mitochondrial membrane, is the location of several critical steps in cellular respiration:
-
Pyruvate oxidation: Pyruvate, the end product of glycolysis (occurring in the cytoplasm), enters the mitochondrial matrix through specific transport proteins. Inside the matrix, pyruvate is converted into acetyl-CoA, releasing carbon dioxide (CO₂) and generating NADH, a crucial electron carrier. This process involves the pyruvate dehydrogenase complex, a large multi-enzyme complex.
-
The Krebs cycle (citric acid cycle): Acetyl-CoA enters the Krebs cycle, a series of eight enzyme-catalyzed reactions that further oxidize the carbon atoms of pyruvate. This cycle generates ATP, NADH, FADH₂ (another electron carrier), and releases carbon dioxide as a byproduct. All the enzymes of the Krebs cycle are soluble and reside within the mitochondrial matrix.
Beyond the Mitochondria: Glycolysis in the Cytoplasm
While the mitochondria are the main engines of ATP production, the process of cellular respiration begins in the cytoplasm with glycolysis. This anaerobic process breaks down glucose into two pyruvate molecules, yielding a small amount of ATP and NADH. This initial step is universal, occurring in both prokaryotes and eukaryotes. The pyruvate molecules then need to be transported into the mitochondria to continue the process of cellular respiration.
The Shuttle Systems: Transporting Reducing Equivalents
The NADH produced during glycolysis in the cytoplasm cannot directly cross the mitochondrial inner membrane. Therefore, specialized shuttle systems, such as the glycerol-3-phosphate shuttle and the malate-aspartate shuttle, are employed to transport the reducing equivalents (electrons) from cytoplasmic NADH into the mitochondrial matrix. These shuttles indirectly transfer electrons to mitochondrial NADH or FADH₂, ensuring that the energy stored in these reducing equivalents can be harnessed during oxidative phosphorylation.
The Electron Transport Chain and Chemiosmosis: Generating the ATP
The electron transport chain (ETC) is a series of protein complexes embedded in the inner mitochondrial membrane. Electrons from NADH and FADH₂, generated during glycolysis, pyruvate oxidation, and the Krebs cycle, are passed along the ETC. This electron flow releases energy, which is used to pump protons (H⁺) from the mitochondrial matrix into the intermembrane space, establishing the crucial proton gradient.
ATP synthase, a remarkable molecular machine also embedded in the inner mitochondrial membrane, harnesses the energy stored in this proton gradient. Protons flow back into the matrix through ATP synthase, driving the synthesis of ATP from ADP and inorganic phosphate (Pi). This process is called chemiosmosis. The majority of ATP generated during cellular respiration is produced through oxidative phosphorylation driven by the proton gradient.
Regulation of Cellular Respiration: A Dynamic Process
Cellular respiration is not a static process; its rate is tightly regulated to meet the energy demands of the cell. Several factors influence the rate of respiration, including:
-
Substrate availability: The availability of glucose and oxygen directly impacts the rate of glycolysis and oxidative phosphorylation, respectively.
-
Enzyme activity: The activity of key enzymes involved in glycolysis, the Krebs cycle, and the electron transport chain can be regulated through allosteric mechanisms and feedback inhibition.
-
Hormonal regulation: Hormones like insulin and glucagon play significant roles in regulating glucose metabolism and, consequently, cellular respiration.
-
Cellular energy levels: The ATP/ADP ratio acts as a sensor, influencing the activity of key enzymes in the pathway.
Variations and Adaptations: Cellular Respiration in Different Eukaryotes
While the fundamental steps of cellular respiration are conserved across eukaryotes, variations exist depending on the organism's metabolic needs and environmental conditions. For instance, some organisms may have alternative electron acceptors in anaerobic conditions, while others may have modified versions of the electron transport chain.
Conclusion: A Symphony of Compartmentalization
Cellular respiration is a remarkable example of cellular organization and efficiency. The compartmentalization within eukaryotic cells, with glycolysis in the cytoplasm and the majority of ATP production within the mitochondria, allows for a highly coordinated and optimized energy production process. The intricate interplay between the different organelles and the sophisticated regulatory mechanisms ensures that the cell's energy needs are met effectively. Further research continues to unravel the intricate details of this fundamental process, providing deeper insights into the complexities of life itself. Understanding the precise location of each step in cellular respiration is not just an academic exercise but also crucial for developing strategies to combat metabolic diseases and improve human health. The mitochondrial function, in particular, is the subject of intense investigation, as its dysfunction is linked to a wide range of human diseases. Thus, a clear understanding of the cellular machinery responsible for energy production remains vital.
Latest Posts
Latest Posts
-
What Is A Rectangular Coordinate System
Apr 15, 2025
-
Where Is The Breathing Center Located
Apr 15, 2025
-
Science Words That Start With J Biology
Apr 15, 2025
-
What Is The Molar Enthalpy Of Fusion
Apr 15, 2025
-
Is A Solid Denser Than A Liquid
Apr 15, 2025
Related Post
Thank you for visiting our website which covers about Where Does Cellular Respiration Take Place In Eukaryotes . We hope the information provided has been useful to you. Feel free to contact us if you have any questions or need further assistance. See you next time and don't miss to bookmark.