Where Does The Energy For Active Transport Come From
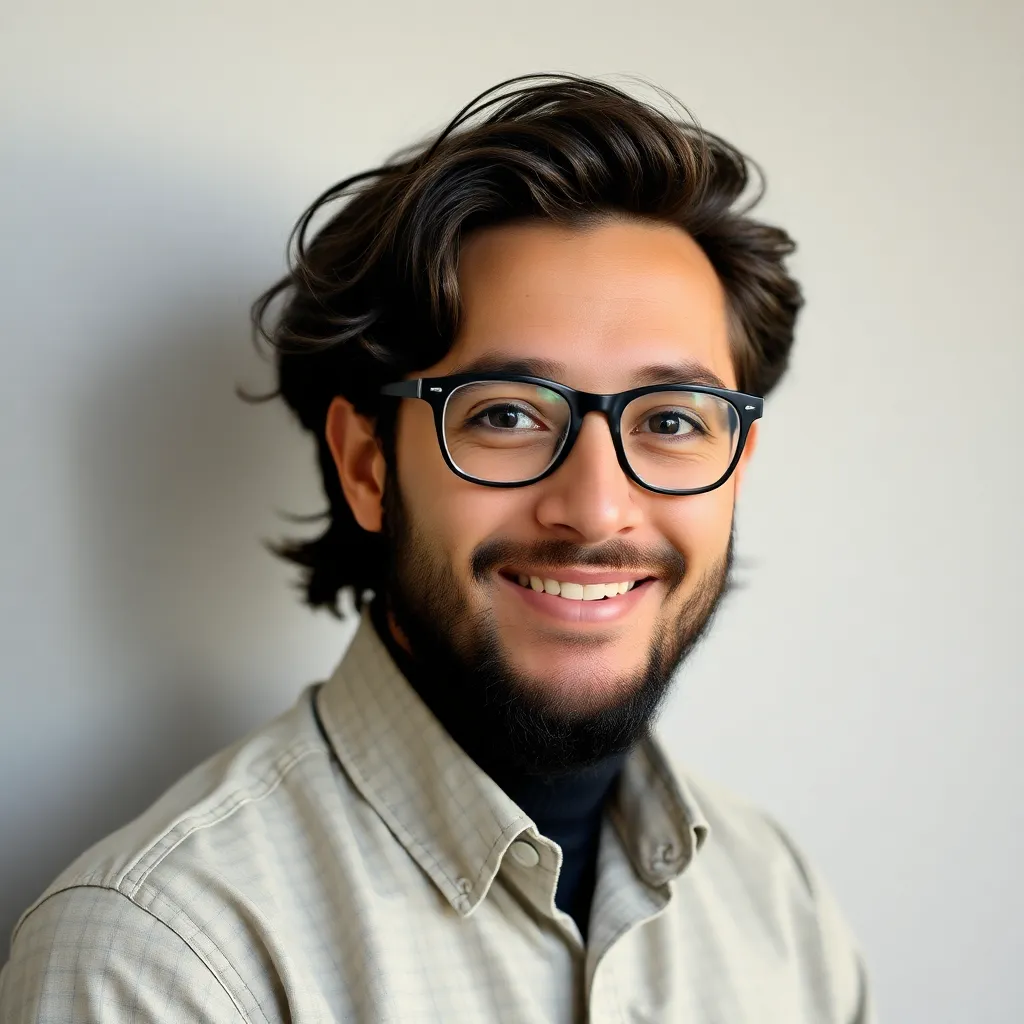
Muz Play
Mar 10, 2025 · 6 min read

Table of Contents
Where Does the Energy for Active Transport Come From?
Active transport, a fundamental process in biology, is the movement of molecules across a cell membrane against their concentration gradient. This means molecules are moved from an area of low concentration to an area of high concentration, a process that requires energy. But where does this energy come from? Understanding this energy source is crucial to grasping the intricacies of cellular function and overall biological processes. This article delves into the various energy sources powering active transport, exploring the underlying mechanisms and their significance in diverse biological systems.
The Crucial Role of ATP: The Cellular Energy Currency
The primary energy source fueling most active transport mechanisms is adenosine triphosphate (ATP). ATP is often referred to as the "energy currency" of the cell because it stores and releases energy in a readily usable form. This energy is stored in the high-energy phosphate bonds connecting the three phosphate groups in the ATP molecule. Hydrolysis of these bonds, breaking them down into adenosine diphosphate (ADP) and inorganic phosphate (Pi), releases a significant amount of energy. This energy is then harnessed to power various cellular processes, including active transport.
How ATP Powers Active Transport: The ATPase Pumps
Many active transport systems utilize ATPase pumps, also known as transporter ATPases. These are transmembrane proteins that bind ATP and undergo conformational changes upon ATP hydrolysis. These conformational changes facilitate the movement of molecules against their concentration gradient. The binding of ATP triggers a series of structural rearrangements in the protein, creating a binding site for the transported molecule on one side of the membrane. The subsequent hydrolysis of ATP induces further conformational changes, resulting in the release of the molecule on the opposite side of the membrane.
Several different types of ATPase pumps exist, each specialized for transporting specific molecules. These include:
-
P-type ATPases: These pumps become phosphorylated during the transport cycle, a crucial step in their mechanism. Examples include the sodium-potassium pump (Na+/K+ ATPase) essential for maintaining cellular ion gradients, and the calcium pump (Ca2+ ATPase) crucial for regulating intracellular calcium levels.
-
V-type ATPases: These pumps utilize ATP hydrolysis to move protons (H+) across membranes, often creating an acidic environment within organelles like lysosomes. Their function is vital in maintaining the acidic pH necessary for lysosomal enzyme activity.
-
F-type ATPases (ATP Synthases): While primarily known for their role in ATP synthesis during oxidative phosphorylation, F-type ATPases can also function in reverse, utilizing ATP hydrolysis to transport protons across membranes. This process contributes to maintaining electrochemical gradients in certain situations.
-
ABC transporters: This large family of transporters utilizes ATP hydrolysis to transport a wide range of molecules, including ions, sugars, amino acids, and even drugs. They are critical for diverse cellular functions, from nutrient uptake to detoxification.
Beyond ATP: Alternative Energy Sources
While ATP is the dominant energy source for active transport, other energy sources can also power specific transport mechanisms. These include:
Ion Gradients: Secondary Active Transport
Secondary active transport, also known as cotransport or coupled transport, utilizes the energy stored in an existing ion gradient, often created by ATP-driven pumps. This means that one molecule is moved against its concentration gradient by harnessing the movement of another molecule down its concentration gradient. The movement of the first molecule down its concentration gradient provides the energy needed for the uphill transport of the second molecule.
For example, the sodium-glucose cotransporter (SGLT) uses the sodium ion gradient (established by the Na+/K+ ATPase) to transport glucose into cells against its concentration gradient. As sodium ions move into the cell down their concentration gradient, they "pull" glucose along with them, thus requiring no direct ATP expenditure for glucose transport. This mechanism is crucial for glucose absorption in the intestines and kidneys.
Light Energy: Bacteriorhodopsin
In certain organisms, particularly halophilic archaea, light energy can directly power active transport. Bacteriorhodopsin, a light-driven proton pump, utilizes the energy of absorbed photons to transport protons across the cell membrane, creating a proton gradient. This gradient is then used to drive other cellular processes, including ATP synthesis and active transport of various molecules.
The Significance of Active Transport: Maintaining Cellular Homeostasis
Active transport plays a pivotal role in maintaining cellular homeostasis, the stable internal environment necessary for cell survival and function. This is achieved by:
-
Maintaining ion gradients: Active transport establishes and maintains crucial ion gradients across cell membranes. These gradients are essential for nerve impulse transmission, muscle contraction, and other physiological processes. The Na+/K+ ATPase, for instance, maintains the electrochemical gradients necessary for these functions.
-
Nutrient uptake: Active transport is crucial for transporting essential nutrients, such as glucose, amino acids, and ions, into cells against their concentration gradients, ensuring adequate supply for cellular metabolism.
-
Waste removal: Active transport is critical for removing metabolic waste products and toxins from cells, preventing their accumulation and potential damage.
-
Maintaining cellular pH: Active transport systems, like V-type ATPases, help regulate the intracellular pH, ensuring an optimal environment for enzyme activity and other cellular processes.
-
Signal transduction: Active transport plays a role in various signaling pathways by moving molecules involved in these pathways across membranes, thus contributing to cellular responses to external stimuli.
Active Transport and Disease: Implications of Dysfunction
Dysfunction of active transport systems can have severe consequences, leading to various diseases. For example:
-
Mutations in the Na+/K+ ATPase: Mutations in the gene encoding the Na+/K+ ATPase can lead to several disorders, including familial hyperaldosteronism and digitalis toxicity.
-
Cystic fibrosis: This genetic disease is caused by mutations in the cystic fibrosis transmembrane conductance regulator (CFTR), an ABC transporter involved in chloride ion transport. Dysfunction of CFTR leads to thick mucus build-up in the lungs and other organs.
-
Cancer: Dysregulation of active transport systems, including various ABC transporters, is implicated in multi-drug resistance in cancer cells, making treatment challenging.
-
Metabolic disorders: Defects in glucose transporters can result in various metabolic disorders affecting glucose uptake and utilization.
Conclusion: A Complex and Vital Process
Active transport, a sophisticated process essential for life, relies primarily on the energy released during ATP hydrolysis. While ATP is the main energy currency, other sources, such as ion gradients and even light energy, can also power specific transport mechanisms. Understanding the diverse energy sources and mechanisms of active transport is crucial to appreciating its vital role in maintaining cellular homeostasis and overall biological function. Moreover, recognizing the implications of active transport dysfunction highlights its significance in health and disease, underscoring the need for ongoing research into its mechanisms and regulation. The intricacies of this process continue to fascinate and challenge researchers, promising further breakthroughs in understanding cellular biology and its impact on human health.
Latest Posts
Latest Posts
-
Which Is Not A Type Of Muscle Tissue
May 09, 2025
-
How To Find The Mass Of The Excess Reactant
May 09, 2025
-
The Terminal Electron Acceptor In Respiration May Be
May 09, 2025
-
Which Of The Following Is A Barrier To Effective Listening
May 09, 2025
-
Presentacion Sin Titulo Presentaciones De Google
May 09, 2025
Related Post
Thank you for visiting our website which covers about Where Does The Energy For Active Transport Come From . We hope the information provided has been useful to you. Feel free to contact us if you have any questions or need further assistance. See you next time and don't miss to bookmark.