Where Is The Energy In Glucose Molecule Stored
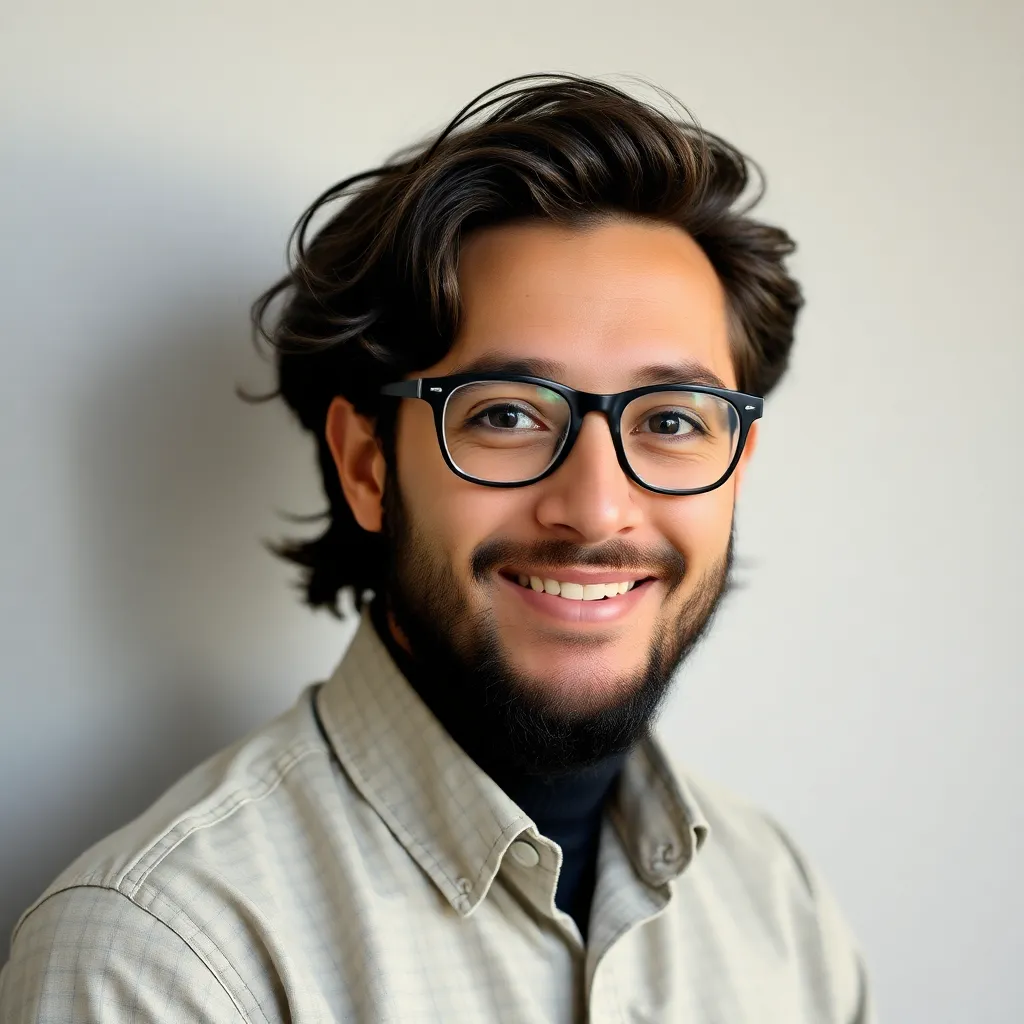
Muz Play
Apr 22, 2025 · 6 min read

Table of Contents
Where is the Energy in a Glucose Molecule Stored? Unlocking the Secrets of Cellular Fuel
Glucose, a simple sugar with the chemical formula C₆H₁₂O₆, is the primary source of energy for most living organisms. But where exactly is this energy stored within its seemingly simple structure? It's not stored as readily available electricity or some easily-accessible chemical potential like a compressed spring. Understanding this requires delving into the intricacies of chemical bonds and the principles of thermodynamics. This article will explore the precise location of glucose's energy, explaining its release through cellular respiration and the crucial role of ATP in powering life's processes.
The Energy Lies Within the Bonds: A Chemical Perspective
The energy in a glucose molecule isn't stored in a single, easily identifiable location. Instead, it's potential energy stored within the chemical bonds connecting its atoms. Specifically, the energy is contained within the covalent bonds between carbon, hydrogen, and oxygen atoms. These bonds represent a relatively stable arrangement, but they hold significant potential energy that can be released through carefully controlled chemical reactions.
High-Energy Bonds: The Key to Understanding Glucose's Energy
The term "high-energy bond" is frequently used in biochemistry to describe bonds that release a significant amount of energy when broken. While not literally "high energy" in terms of bond strength, these bonds are associated with large negative changes in free energy (ΔG) during hydrolysis—a reaction involving the breaking of a bond by adding a water molecule. In glucose, several bonds are considered "high-energy" in this context, particularly those involving the carbon-hydrogen and carbon-oxygen bonds within the molecule's structure.
These bonds are considered high-energy due to a few key factors:
- Electron arrangement: The electrons in these bonds are arranged in a less stable configuration compared to the products formed after bond breakage. This inherent instability contributes to the potential energy release.
- Resonance stabilization: In some cases, the products of bond breakage can exhibit resonance stabilization, a phenomenon where electrons are delocalized over multiple atoms, resulting in a more stable and lower-energy state. This difference in energy contributes to the energy released during bond cleavage.
- Electrostatic interactions: The products of bond breakage may possess more favorable electrostatic interactions compared to the reactant molecule, further contributing to the overall energy released.
Cellular Respiration: Harvesting Energy from Glucose
The energy stored in glucose's chemical bonds isn't directly usable by the cell. Instead, it's harnessed through a series of carefully controlled enzymatic reactions known as cellular respiration. This process occurs in several stages:
1. Glycolysis: Initial Breakdown in the Cytoplasm
Glycolysis, the first stage, takes place in the cytoplasm and doesn't require oxygen. It involves a series of ten enzyme-catalyzed steps that break down glucose into two molecules of pyruvate. During glycolysis, a small amount of ATP (adenosine triphosphate), the cell's primary energy currency, is generated directly through substrate-level phosphorylation. This process involves the direct transfer of a phosphate group from a substrate molecule to ADP (adenosine diphosphate), forming ATP. Additionally, NADH (nicotinamide adenine dinucleotide), an electron carrier, is also produced.
2. Pyruvate Oxidation: Preparing for the Krebs Cycle
Pyruvate, the product of glycolysis, is transported into the mitochondria, the cell's powerhouses. Here, it undergoes oxidative decarboxylation, losing a carbon dioxide molecule and becoming acetyl-CoA. In this step, more NADH is produced.
3. Krebs Cycle (Citric Acid Cycle): Central Metabolic Hub
The acetyl-CoA enters the Krebs cycle, a cyclical series of reactions that further oxidizes the carbon atoms, releasing carbon dioxide as a byproduct. This cycle generates a small amount of ATP through substrate-level phosphorylation, but its main role is to produce large quantities of NADH and FADH₂ (flavin adenine dinucleotide), another electron carrier. These molecules are crucial for the next stage.
4. Oxidative Phosphorylation: The Powerhouse of ATP Production
Oxidative phosphorylation occurs in the inner mitochondrial membrane and involves two key processes:
- Electron transport chain: The electrons carried by NADH and FADH₂ are passed down a series of protein complexes embedded in the inner mitochondrial membrane. As electrons move down the chain, energy is released, creating a proton gradient across the membrane.
- Chemiosmosis: The proton gradient generated by the electron transport chain drives ATP synthesis through ATP synthase, an enzyme that uses the flow of protons back across the membrane to phosphorylate ADP to ATP. This is oxidative phosphorylation, generating the vast majority of ATP produced during cellular respiration. This process is far more efficient than substrate-level phosphorylation, producing a significantly higher ATP yield.
ATP: The Universal Energy Currency
The energy released during the breakdown of glucose is not directly used to power cellular processes. Instead, it's efficiently captured and stored in the high-energy phosphate bonds of ATP. ATP is often described as the cell's "energy currency" because it acts as an intermediary, transferring energy from energy-releasing processes (like cellular respiration) to energy-requiring processes (like muscle contraction, protein synthesis, and active transport). The energy stored in the phosphate bonds of ATP is readily available for cellular work.
The hydrolysis of ATP to ADP and inorganic phosphate (Pi) releases a significant amount of energy, driving endergonic (energy-requiring) reactions:
ATP + H₂O → ADP + Pi + Energy
Beyond Glucose: Other Energy Sources
While glucose is the primary energy source, organisms can utilize other molecules for energy production. These include:
- Fatty acids: These are broken down through beta-oxidation, generating acetyl-CoA which enters the Krebs cycle. Fatty acids provide a significantly higher energy yield compared to glucose.
- Amino acids: These can be converted into various intermediates of the Krebs cycle, contributing to ATP production.
- Ketone bodies: These are produced during periods of fasting or low carbohydrate intake and can be used as an alternative energy source.
The energy stored in these molecules, similar to glucose, resides in their chemical bonds and is harnessed through various metabolic pathways.
Conclusion: A Complex Network of Energy Transfer
The energy stored within a glucose molecule is not localized to a specific point but is distributed throughout its chemical bonds. The breakdown of glucose through cellular respiration carefully extracts this energy, converting it into the readily usable form of ATP. This process is a highly intricate and efficient system, enabling life's diverse energy-demanding functions. Understanding the location and harnessing of energy within glucose and other fuel molecules is fundamental to comprehending the basic principles of biochemistry and the remarkable complexity of life itself. Further research continually expands our knowledge of metabolic pathways and their regulation, further refining our understanding of this crucial energy transfer system.
Latest Posts
Latest Posts
-
Myoglobin And The Subunits Of Hemoglobin Have
Apr 22, 2025
-
Mass Moment Of Inertia Of A Rod
Apr 22, 2025
-
Which Change Of State Involves A Release Of Energy
Apr 22, 2025
-
How To Write A System Of Linear Equations
Apr 22, 2025
-
What Type Of Rock Contains Large Crystals
Apr 22, 2025
Related Post
Thank you for visiting our website which covers about Where Is The Energy In Glucose Molecule Stored . We hope the information provided has been useful to you. Feel free to contact us if you have any questions or need further assistance. See you next time and don't miss to bookmark.