Which Monomer Units Combine To Form Nucleic Acids
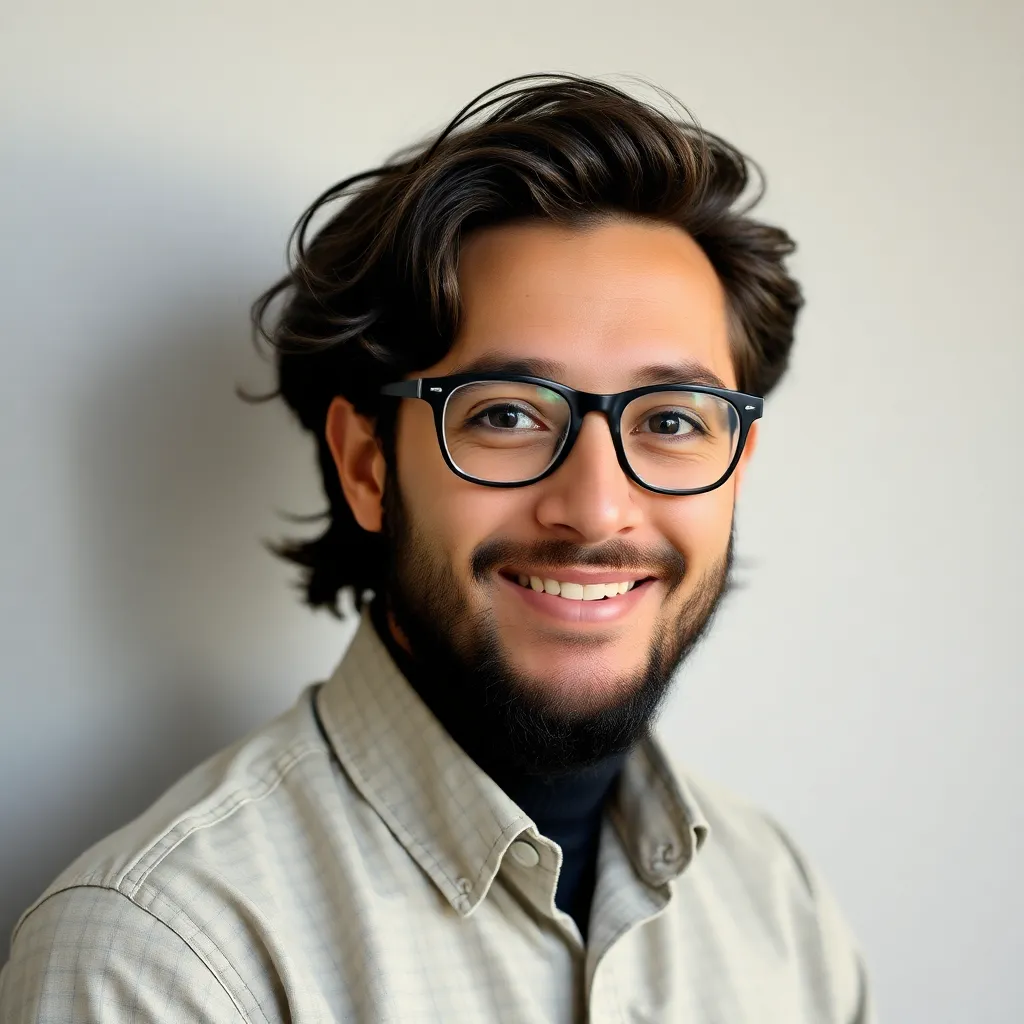
Muz Play
May 09, 2025 · 6 min read
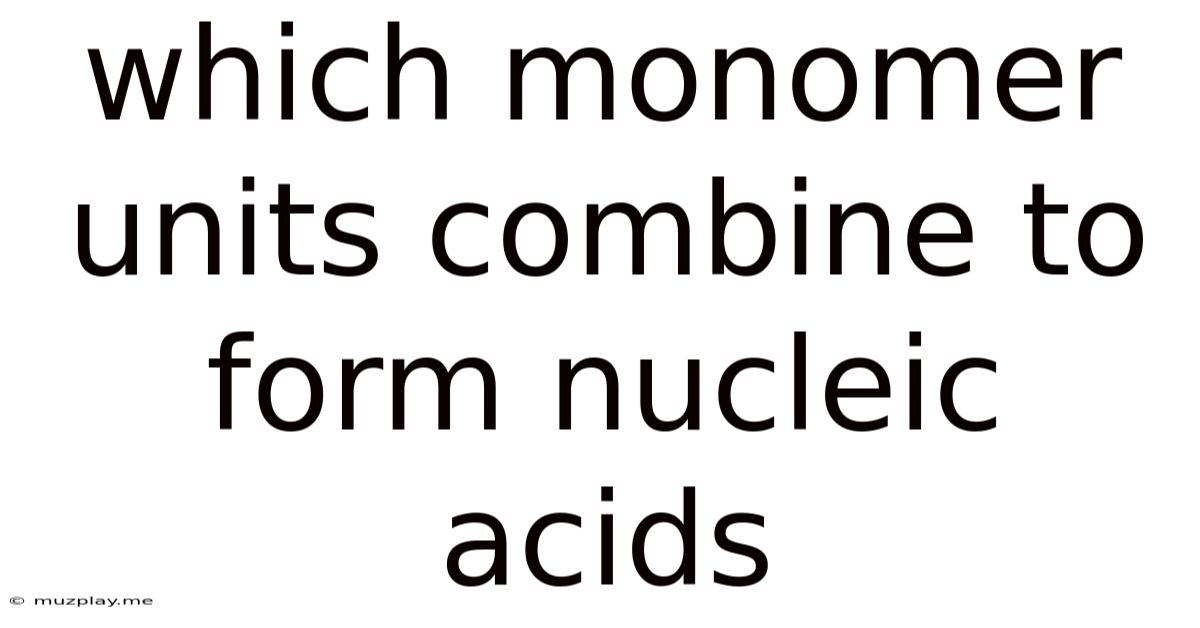
Table of Contents
Which Monomer Units Combine to Form Nucleic Acids?
Nucleic acids are fundamental macromolecules crucial for life, acting as the blueprints for biological organisms and playing vital roles in protein synthesis and cellular regulation. Understanding their structure, specifically the monomer units that combine to form them, is paramount to grasping their function and significance. This comprehensive article delves into the intricacies of nucleic acid structure, focusing on the monomers that assemble to create these essential biopolymers.
The Building Blocks: Nucleotides
The monomer units that form nucleic acids are nucleotides. These aren't simply strung together randomly; the sequence of nucleotides dictates the genetic information encoded within the nucleic acid. Each nucleotide is composed of three key components:
1. A Pentose Sugar: The Backbone's Foundation
The backbone of a nucleic acid strand is formed by a chain of sugar molecules. There are two types of pentose (five-carbon) sugars found in nucleotides:
- Ribose: Found in ribonucleic acid (RNA). Ribose has a hydroxyl (-OH) group attached to the 2' carbon atom.
- Deoxyribose: Found in deoxyribonucleic acid (DNA). Deoxyribose lacks the hydroxyl group at the 2' carbon, instead having only a hydrogen atom (-H). This seemingly minor difference significantly impacts the stability and function of the two nucleic acids.
The difference in the 2'-carbon atom is critical. The presence of the hydroxyl group in ribose makes RNA more reactive and less stable than DNA. This increased reactivity contributes to RNA's diverse roles in gene expression and regulation, but also its shorter lifespan compared to the more stable DNA.
2. A Nitrogenous Base: The Information Carriers
Attached to the 1' carbon of the pentose sugar is a nitrogenous base. These bases are heterocyclic organic molecules containing nitrogen atoms, and they are classified into two groups based on their structure:
- Purines: These are double-ringed structures. The two purine bases found in nucleic acids are adenine (A) and guanine (G).
- Pyrimidines: These are single-ringed structures. The pyrimidine bases are cytosine (C), thymine (T), and uracil (U). Thymine is found exclusively in DNA, while uracil is found only in RNA.
The sequence of these nitrogenous bases along the nucleic acid strand represents the genetic code. The specific order of A, T, C, and G (in DNA) or A, U, C, and G (in RNA) determines the genetic information. This order directs the synthesis of proteins and influences various cellular processes.
3. A Phosphate Group: Linking the Units
The third component of a nucleotide is a phosphate group (PO₄³⁻). This negatively charged group is attached to the 5' carbon of the pentose sugar. The phosphate group plays a crucial role in linking nucleotides together to form the polynucleotide chain.
The Formation of the Nucleic Acid Chain: Phosphodiester Bonds
Nucleotides are joined together by phosphodiester bonds. This bond forms between the phosphate group of one nucleotide and the 3' hydroxyl group of the sugar in the adjacent nucleotide. This creates a sugar-phosphate backbone that runs along the length of the nucleic acid strand. The directionality of this backbone is crucial; it is always described as having a 5' end (where the phosphate group is free) and a 3' end (where the hydroxyl group is free). This 5' to 3' directionality is critical during DNA replication and transcription.
The process of forming a phosphodiester bond involves a dehydration reaction (also known as a condensation reaction). A molecule of water is removed as the bond is formed between the phosphate group and the hydroxyl group. This process is catalyzed by enzymes, ensuring precise and accurate joining of nucleotides.
DNA: The Double Helix
Deoxyribonucleic acid (DNA) is a double-stranded helix, meaning two polynucleotide strands are intertwined. These strands run antiparallel to each other, meaning that one strand runs in the 5' to 3' direction while the other runs in the 3' to 5' direction. The two strands are held together by hydrogen bonds between the nitrogenous bases.
Base pairing rules are fundamental to DNA structure and function. Adenine (A) always pairs with thymine (T) through two hydrogen bonds, while guanine (G) always pairs with cytosine (C) through three hydrogen bonds. This specific base pairing ensures the complementary nature of the two DNA strands, allowing for accurate replication and transcription. The double helix structure provides stability and protects the genetic information within.
The double helix is not a rigid structure; it can undergo conformational changes depending on environmental factors such as temperature and ionic strength. These changes are important in regulating gene expression and DNA replication.
RNA: The Versatile Single Strand
Ribonucleic acid (RNA) is typically single-stranded, although it can fold into complex secondary and tertiary structures due to base pairing within the same molecule. The absence of a second strand contributes to RNA's greater flexibility and versatility compared to DNA. RNA molecules participate in a variety of crucial cellular processes:
- Messenger RNA (mRNA): Carries genetic information from DNA to ribosomes for protein synthesis.
- Transfer RNA (tRNA): Brings amino acids to the ribosomes during protein synthesis.
- Ribosomal RNA (rRNA): Forms part of the ribosome structure and participates in protein synthesis.
- Small nuclear RNA (snRNA): Involved in RNA splicing.
- MicroRNA (miRNA): Regulates gene expression.
While RNA primarily uses uracil (U) instead of thymine (T), the base pairing rules are similar, with A pairing with U and G pairing with C. The single-stranded nature of RNA allows for complex intramolecular interactions, leading to diverse structures and functions.
Differences Between DNA and RNA: A Summary
Feature | DNA | RNA |
---|---|---|
Sugar | Deoxyribose | Ribose |
Structure | Double-stranded helix | Typically single-stranded |
Bases | A, T, C, G | A, U, C, G |
Stability | More stable | Less stable |
Location | Primarily in the nucleus | Nucleus and cytoplasm |
Function | Stores genetic information | Diverse roles in gene expression |
The Significance of Nucleotide Sequence
The precise order of nucleotides in a nucleic acid strand determines its function. This sequence encodes the genetic information that dictates the characteristics of an organism and directs cellular processes. Changes in the sequence, such as mutations, can have profound effects on an organism's phenotype.
Understanding the monomer units of nucleic acids and how they are assembled into polynucleotide chains is fundamental to comprehending the mechanisms of inheritance, protein synthesis, gene regulation, and many other vital biological processes. The detailed structure and function of DNA and RNA are testament to the elegance and efficiency of life's fundamental building blocks. Future research continues to unravel the intricacies of nucleic acid structure and function, paving the way for advancements in medicine, biotechnology, and our understanding of life itself. The precise arrangement of these seemingly simple monomer units underlies the complexity of life.
Latest Posts
Latest Posts
-
How Are Dispersion Forces Similar To Dipole Dipole Interactions
May 10, 2025
-
Differentiate Between Density Dependent And Density Independent Limiting Factors
May 10, 2025
-
Find The Temperature Of An Unidentified Substance
May 10, 2025
-
Magnetic Fields Always Exist In What Direction
May 10, 2025
-
Difference Between Cladogram And Phylogenetic Tree
May 10, 2025
Related Post
Thank you for visiting our website which covers about Which Monomer Units Combine To Form Nucleic Acids . We hope the information provided has been useful to you. Feel free to contact us if you have any questions or need further assistance. See you next time and don't miss to bookmark.