Which Monomer Units Combine To Form Proteins
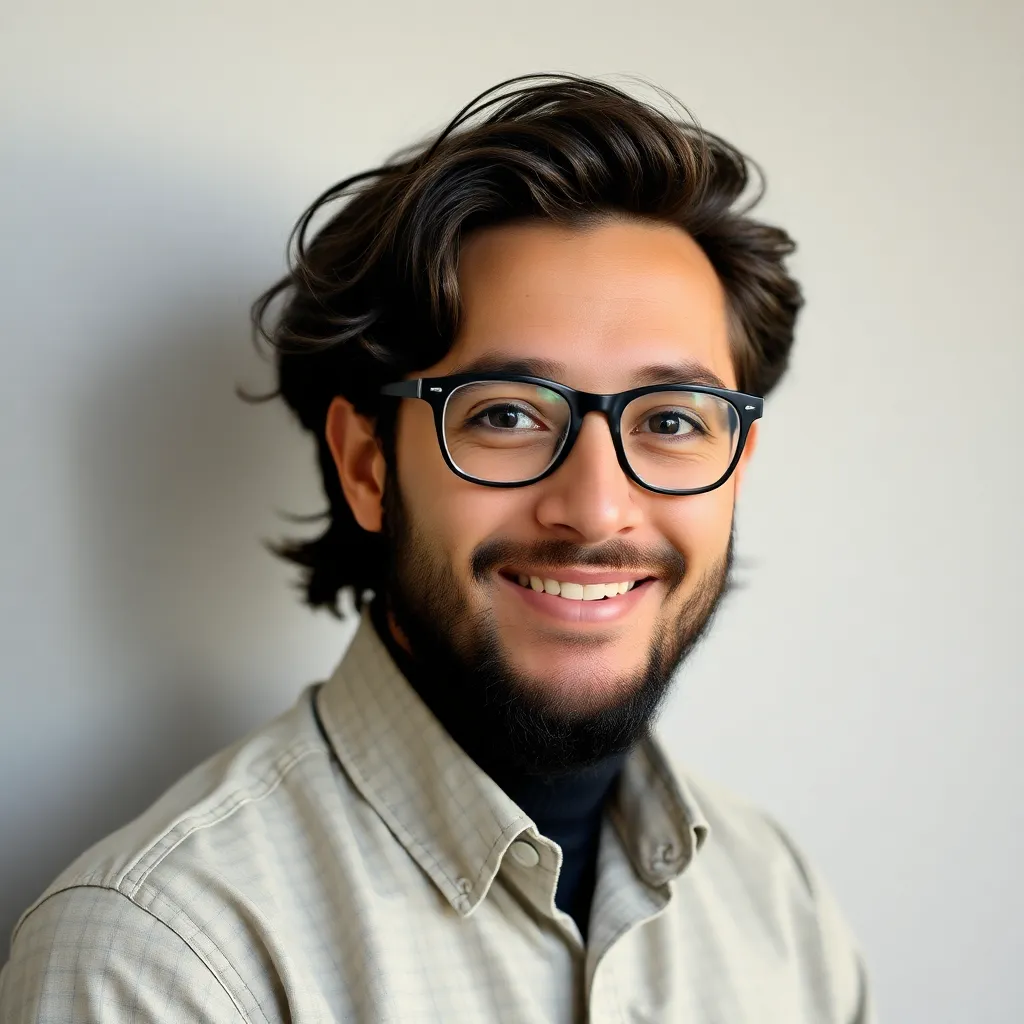
Muz Play
Mar 11, 2025 · 6 min read

Table of Contents
Which Monomer Units Combine to Form Proteins?
Proteins are the workhorses of the cell, carrying out a vast array of functions crucial for life. From catalyzing biochemical reactions as enzymes to providing structural support and facilitating cell signaling, their roles are indispensable. But what are these complex molecules fundamentally made of? The answer lies in their monomeric building blocks: amino acids.
Understanding Amino Acids: The Building Blocks of Proteins
Amino acids are organic molecules that serve as the fundamental units of proteins. They're characterized by a specific structure containing four key components:
-
A central carbon atom (α-carbon): This carbon atom acts as the backbone, connecting the other three components.
-
An amino group (-NH₂): This is a basic group, meaning it can accept a proton (H⁺). It's responsible for the amino acid's basic properties.
-
A carboxyl group (-COOH): This is an acidic group, capable of donating a proton (H⁺). It gives the amino acid its acidic properties.
-
A variable side chain (R-group): This is what differentiates one amino acid from another. The R-group can be anything from a simple hydrogen atom (as in glycine) to a complex aromatic ring structure (as in tryptophan). The properties of the R-group—size, charge, polarity, and hydrophobicity—significantly influence the protein's overall structure and function.
The Diversity of Amino Acids
There are 20 standard amino acids that are genetically coded and commonly found in proteins. These amino acids can be categorized based on several properties of their R-groups:
1. Based on Polarity:
-
Nonpolar (hydrophobic) amino acids: These amino acids have R-groups that are hydrophobic, meaning they repel water. They tend to cluster together in the interior of proteins, away from the aqueous environment. Examples include alanine, valine, leucine, isoleucine, methionine, phenylalanine, tryptophan, and proline. Proline is unique because its R-group is bonded to both the α-carbon and the amino group, forming a ring structure that restricts its flexibility.
-
Polar (hydrophilic) amino acids: These amino acids have R-groups that are hydrophilic, meaning they attract water. They often reside on the protein's surface, interacting with the surrounding water molecules. These can be further categorized into:
- Uncharged polar amino acids: These amino acids possess polar R-groups but do not carry a net charge at physiological pH. Examples include serine, threonine, cysteine, tyrosine, asparagine, and glutamine. Cysteine is unique due to its sulfhydryl (-SH) group, which can form disulfide bonds with other cysteine residues, contributing to protein stability.
- Charged polar amino acids: These amino acids have R-groups that carry a net charge at physiological pH. They can be either positively charged (basic) or negatively charged (acidic).
- Basic amino acids: These possess positively charged R-groups at physiological pH. Examples include lysine, arginine, and histidine.
- Acidic amino acids: These possess negatively charged R-groups at physiological pH. Examples include aspartic acid and glutamic acid.
2. Based on Essential vs. Non-Essential:
The human body can synthesize some amino acids, while others must be obtained through the diet.
-
Essential amino acids: These cannot be synthesized by the body and must be obtained from food. They include histidine, isoleucine, leucine, lysine, methionine, phenylalanine, threonine, tryptophan, and valine.
-
Non-essential amino acids: These can be synthesized by the body. They include alanine, arginine, asparagine, aspartic acid, cysteine, glutamic acid, glutamine, glycine, proline, serine, and tyrosine.
Peptide Bonds: Linking Amino Acids
Amino acids combine to form proteins through peptide bonds. This is a covalent bond formed between the carboxyl group (-COOH) of one amino acid and the amino group (-NH₂) of another amino acid. During peptide bond formation, a molecule of water is released (a dehydration reaction). The resulting linkage is an amide bond, specifically termed a peptide bond in the context of proteins.
Polypeptide Chains: Sequences of Amino Acids
The chain of amino acids linked by peptide bonds is called a polypeptide chain. The sequence of amino acids in a polypeptide chain is determined by the genetic code carried in DNA. This sequence is crucial because it dictates the protein's three-dimensional structure and, ultimately, its function. The amino acid at one end of the polypeptide chain retains a free amino group (N-terminus), while the other end retains a free carboxyl group (C-terminus).
Protein Structure: From Primary to Quaternary
The functional properties of a protein are intimately linked to its three-dimensional structure. This structure can be described at four levels of organization:
1. Primary Structure: This is the linear sequence of amino acids in the polypeptide chain. It's like the blueprint for the protein, dictating all subsequent levels of structure. Even a single amino acid change can drastically alter the protein's function (e.g., sickle cell anemia).
2. Secondary Structure: This refers to local folding patterns within the polypeptide chain, stabilized by hydrogen bonds between the backbone atoms. Common secondary structure elements include:
-
α-helices: Right-handed coiled structures stabilized by hydrogen bonds between the carbonyl oxygen of one amino acid and the amide hydrogen of an amino acid four residues down the chain.
-
β-sheets: Extended, planar structures formed by hydrogen bonds between adjacent polypeptide chains (parallel or antiparallel).
3. Tertiary Structure: This describes the overall three-dimensional arrangement of a polypeptide chain, encompassing all secondary structure elements. It's stabilized by a variety of interactions, including:
-
Hydrophobic interactions: Nonpolar side chains cluster together in the protein's interior, away from water.
-
Hydrogen bonds: These form between polar side chains.
-
Ionic bonds (salt bridges): These occur between oppositely charged side chains.
-
Disulfide bonds: Covalent bonds formed between cysteine residues.
4. Quaternary Structure: This refers to the arrangement of multiple polypeptide chains (subunits) in a multimeric protein. Not all proteins have quaternary structure; some exist as single polypeptide chains. Interactions similar to those stabilizing tertiary structure (hydrophobic interactions, hydrogen bonds, ionic bonds, disulfide bonds) hold the subunits together.
Post-Translational Modifications: Fine-Tuning Protein Function
After synthesis, many proteins undergo post-translational modifications. These modifications alter the protein's structure, function, or stability. Examples include:
-
Glycosylation: The addition of sugar molecules.
-
Phosphorylation: The addition of phosphate groups.
-
Acetylation: The addition of acetyl groups.
-
Ubiquitination: The addition of ubiquitin molecules, often targeting proteins for degradation.
These modifications are crucial for regulating protein activity, localization, and lifespan.
Conclusion: The Intricate World of Protein Structure and Function
The formation of proteins from amino acid monomers is a remarkable example of biological complexity. The precise sequence of amino acids, dictated by the genetic code, determines the protein's unique three-dimensional structure. This structure, in turn, dictates the protein's function, enabling it to perform its myriad roles in the cell. Understanding the interplay between amino acid structure, peptide bond formation, and protein folding is crucial for comprehending the fundamental processes of life. Further research continues to unveil the intricacies of protein structure, function, and regulation, promising advances in fields ranging from medicine to biotechnology. The 20 amino acids, seemingly simple building blocks, combine in countless ways to create the incredible diversity and functionality of proteins, showcasing the elegance and efficiency of biological systems. The study of proteins is a continually evolving field, with new discoveries constantly expanding our understanding of these essential molecules. From investigating protein misfolding in diseases to engineering novel proteins with desired properties, the exploration of the building blocks of life remains a dynamic and rewarding area of research.
Latest Posts
Latest Posts
-
How Many Orbitals In S Subshell
May 09, 2025
-
Why Is Energy Required For The Boiling Process
May 09, 2025
-
Density Of Water At 19 Degrees Celsius
May 09, 2025
-
Compounds Composed Of Cations And Anions
May 09, 2025
-
How Many Atoms Are In 1 00 Moles Of He
May 09, 2025
Related Post
Thank you for visiting our website which covers about Which Monomer Units Combine To Form Proteins . We hope the information provided has been useful to you. Feel free to contact us if you have any questions or need further assistance. See you next time and don't miss to bookmark.