Why Can't Tertiary Alcohols Be Oxidized
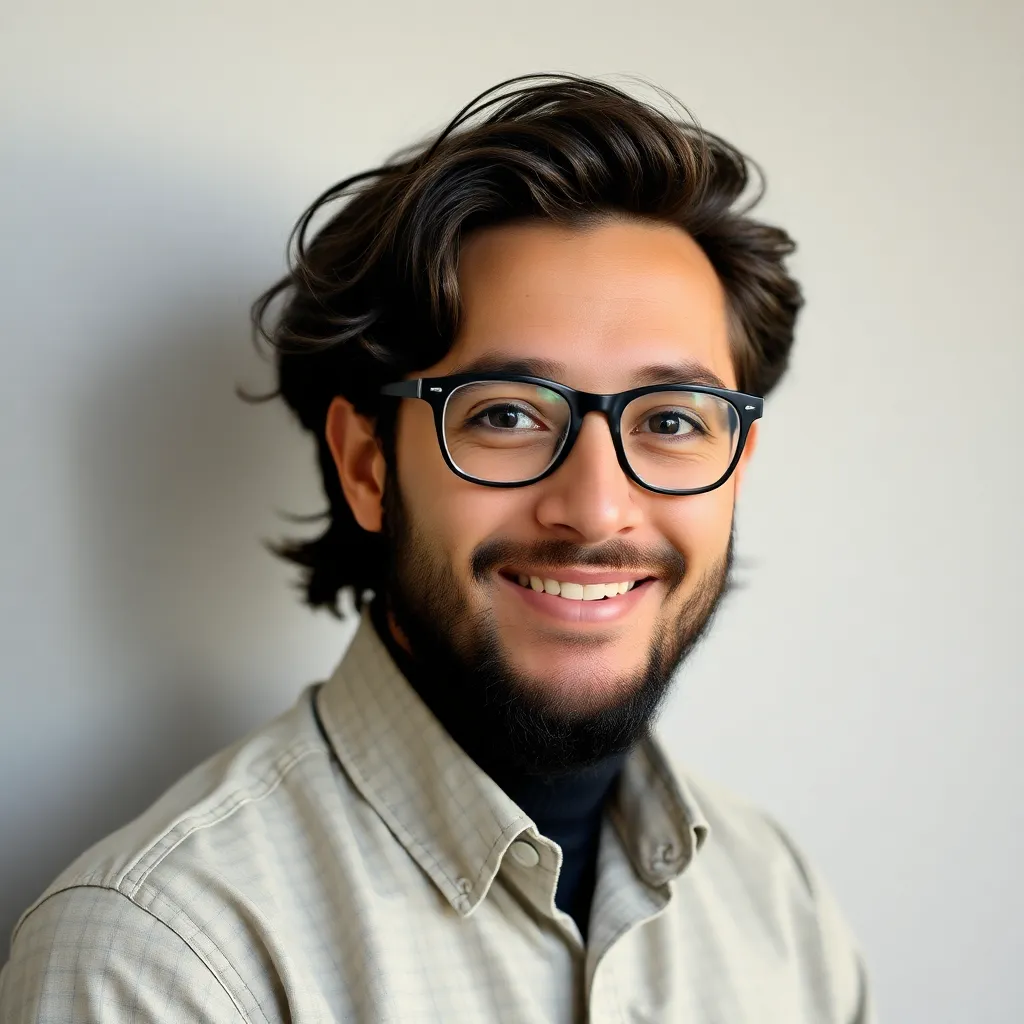
Muz Play
Mar 13, 2025 · 5 min read

Table of Contents
Why Can't Tertiary Alcohols Be Oxidized? A Deep Dive into Organic Chemistry
Tertiary alcohols, unlike their primary and secondary counterparts, stubbornly resist oxidation. This seemingly simple fact hides a rich tapestry of organic chemistry principles, involving reaction mechanisms, molecular structures, and the very nature of oxidation itself. This article will delve deep into the reasons behind this resistance, exploring the underlying chemistry and providing a comprehensive understanding of why tertiary alcohols remain untouched by common oxidizing agents.
Understanding Oxidation in Organic Chemistry
Before we delve into the specifics of tertiary alcohols, let's establish a firm understanding of what oxidation means in the context of organic chemistry. Oxidation, in its simplest form, refers to the loss of electrons or an increase in oxidation state. In organic molecules, this often manifests as:
- Gain of oxygen atoms: Adding an oxygen atom to a carbon atom increases its oxidation state.
- Loss of hydrogen atoms: Removing hydrogen atoms from a carbon atom also increases its oxidation state.
These changes are often coupled; for instance, the oxidation of a primary alcohol to an aldehyde involves the loss of two hydrogen atoms and the addition of one oxygen atom (forming a double bond with the carbon).
Common oxidizing agents used in organic chemistry include potassium dichromate (K₂Cr₂O₇), potassium permanganate (KMnO₄), and chromic acid (H₂CrO₄). These agents are powerful electron acceptors, readily oxidizing susceptible molecules.
The Structure of Tertiary Alcohols: The Key to Inertia
The key to understanding why tertiary alcohols resist oxidation lies in their unique structure. Unlike primary and secondary alcohols, tertiary alcohols lack a hydrogen atom bonded to the carbon atom bearing the hydroxyl (-OH) group. This seemingly minor detail is crucial.
Let's visualize this:
- Primary Alcohol: R-CH₂-OH (R represents an alkyl group) – Has two hydrogen atoms on the carbon bonded to the -OH group.
- Secondary Alcohol: R₁-CH(OH)-R₂ (R₁ and R₂ represent alkyl groups) – Has one hydrogen atom on the carbon bonded to the -OH group.
- Tertiary Alcohol: R₁-C(OH)-R₂R₃ (R₁, R₂, and R₃ represent alkyl groups) – Has zero hydrogen atoms on the carbon bonded to the -OH group.
This absence of a hydrogen atom on the alpha-carbon (the carbon directly attached to the hydroxyl group) is the primary reason tertiary alcohols cannot be oxidized using typical oxidizing agents.
The Mechanism of Alcohol Oxidation: A Comparative Analysis
The oxidation of primary and secondary alcohols proceeds through a series of steps involving the formation of a chromate ester intermediate. This intermediate undergoes elimination of a proton (H⁺) from the alpha-carbon, leading to the formation of a carbonyl compound (aldehyde or ketone). Let's examine this mechanism:
1. Formation of Chromate Ester: The alcohol reacts with the oxidizing agent (e.g., chromic acid) to form a chromate ester.
2. Proton Elimination: A proton (H⁺) is eliminated from the alpha-carbon, leading to the formation of a carbon-oxygen double bond.
3. Reduction of the Oxidizing Agent: The oxidizing agent is reduced, gaining electrons in the process.
Why this mechanism fails for tertiary alcohols: Tertiary alcohols lack the crucial alpha-hydrogen atom required for the proton elimination step (step 2). Without this hydrogen, the chromate ester intermediate cannot undergo the necessary rearrangement to form a carbonyl compound. The reaction essentially stalls, leaving the tertiary alcohol unchanged.
Alternative Oxidation Pathways: Breaking the Rules?
While direct oxidation to a carbonyl compound is impossible, it's important to note that tertiary alcohols can undergo other types of oxidation reactions under specific conditions. These reactions, however, don't involve the typical mechanisms discussed above and often lead to different products. Examples include:
- Degradation Reactions: Under harsh conditions, tertiary alcohols can undergo degradation reactions, breaking down into smaller molecules. These reactions are usually not considered "simple oxidations" in the same sense as the oxidation of primary and secondary alcohols.
- Oxidative Cleavage: Specific reagents and conditions can induce oxidative cleavage of the carbon-carbon bonds adjacent to the tertiary alcohol group. This leads to the formation of carbonyl compounds, but the process is significantly different from the direct oxidation of primary or secondary alcohols. It's crucial to understand that this is not a direct oxidation of the alcohol group itself, but rather a breakdown of the molecule around it.
Practical Implications and Applications
The inability of tertiary alcohols to undergo simple oxidation has significant implications in various fields:
- Synthetic Organic Chemistry: Chemists leverage this property when designing synthetic routes. The stability of tertiary alcohols under typical oxidizing conditions is exploited to protect functional groups or to selectively oxidize other alcohols in a molecule.
- Industrial Processes: This characteristic plays a role in industrial processes involving alcohol oxidation. The selective oxidation of primary and secondary alcohols, while leaving tertiary alcohols untouched, is crucial in various manufacturing scenarios.
- Biological Systems: The resistance of tertiary alcohols to oxidation is relevant in biological systems, where selective oxidation-reduction reactions are vital for metabolic pathways.
Distinguishing Tertiary Alcohols: Analytical Techniques
Identifying tertiary alcohols often requires a combination of techniques:
- Spectroscopy: Nuclear Magnetic Resonance (NMR) spectroscopy can be used to distinguish tertiary alcohols based on the chemical shift of the carbon atom attached to the hydroxyl group and the absence of alpha-hydrogens. Infrared (IR) spectroscopy can also provide information about the presence of the hydroxyl group.
- Chemical Tests: While there isn't a definitive single test, chemical methods like the Lucas test (which differentiates between primary, secondary, and tertiary alcohols based on reaction rates) can indirectly help in identification. However, this is not specific only to tertiary alcohols.
- Chromatography: Techniques like Gas Chromatography (GC) and High-Performance Liquid Chromatography (HPLC) can be used to separate and identify different alcohol types based on their physical properties.
Conclusion: A Cornerstone of Organic Chemistry Understanding
The inability of tertiary alcohols to be oxidized using standard methods is not a quirk but a direct consequence of their molecular structure. This fundamental concept highlights the crucial relationship between molecular structure, reaction mechanisms, and the outcomes of chemical transformations. Understanding this principle is foundational for advanced study in organic chemistry and crucial for applications ranging from synthetic organic chemistry to industrial processes and the study of biological systems. The apparent simplicity of the question "Why can't tertiary alcohols be oxidized?" belies the wealth of underlying chemical principles it encompasses.
Latest Posts
Latest Posts
-
How Many Orbitals In S Subshell
May 09, 2025
-
Why Is Energy Required For The Boiling Process
May 09, 2025
-
Density Of Water At 19 Degrees Celsius
May 09, 2025
-
Compounds Composed Of Cations And Anions
May 09, 2025
-
How Many Atoms Are In 1 00 Moles Of He
May 09, 2025
Related Post
Thank you for visiting our website which covers about Why Can't Tertiary Alcohols Be Oxidized . We hope the information provided has been useful to you. Feel free to contact us if you have any questions or need further assistance. See you next time and don't miss to bookmark.