Why Does Each Element Have A Unique Spectrum
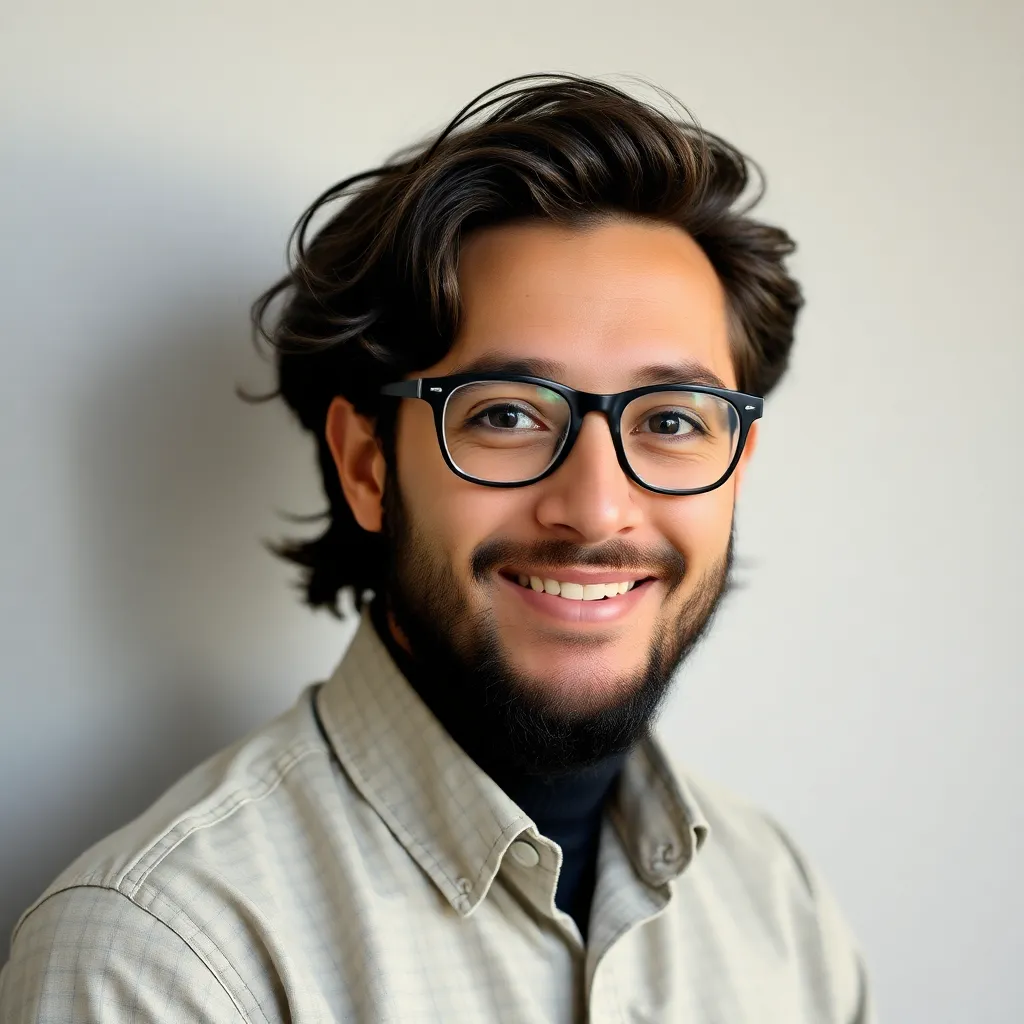
Muz Play
Apr 27, 2025 · 6 min read

Table of Contents
Why Does Each Element Have a Unique Spectrum?
The universe is a kaleidoscope of light, and within that light lies a hidden code: the unique spectral fingerprint of each element. Understanding why each element boasts a distinctive spectrum is fundamental to many scientific disciplines, from astronomy to analytical chemistry. This seemingly simple question leads us down a fascinating rabbit hole exploring the quantum nature of atoms and the interaction of light and matter.
The Quantum Leap: Atomic Structure and Energy Levels
At the heart of this phenomenon lies the quantum mechanical model of the atom. Unlike the classical model of a miniature solar system, the quantum model depicts electrons orbiting the nucleus not in neat, predictable paths, but rather in specific energy levels or shells. These energy levels are quantized, meaning electrons can only exist in these discrete energy states and nowhere in between. Think of it like a staircase: you can stand on a specific step, but not halfway between steps.
Quantized Energy and Electron Transitions
The electrons within an atom are confined to these specific energy levels. The lowest energy level, closest to the nucleus, is called the ground state. However, electrons can absorb energy from external sources, such as heat or light, causing them to jump to a higher energy level – an excited state. This transition is not continuous; it requires a precise amount of energy, corresponding to the energy difference between the two levels.
The Instability of Excited States
Excited states are inherently unstable. The electron will spontaneously return to a lower energy level, releasing the absorbed energy in the form of a photon, a particle of light. The energy of this photon is exactly equal to the energy difference between the initial and final energy levels of the electron. This is the key to understanding unique spectral fingerprints.
The Birth of a Spectral Line: Wavelength and Frequency
The energy of a photon is directly related to its frequency (ν) and wavelength (λ) through the equation E = hν = hc/λ, where h is Planck's constant and c is the speed of light. Because the energy differences between electron energy levels are unique to each element, the photons emitted during electron transitions also possess unique energies, frequencies, and wavelengths.
The Electromagnetic Spectrum
These photons, with their unique wavelengths, fall into different regions of the electromagnetic spectrum. Visible light is only a small portion of this spectrum, encompassing wavelengths roughly between 400 nm (violet) and 700 nm (red). Other regions include ultraviolet (shorter wavelengths), infrared (longer wavelengths), radio waves, microwaves, X-rays, and gamma rays. The specific wavelengths of light emitted by an element determine its unique emission spectrum.
Unique Spectral Fingerprints: Why No Two are Alike?
The uniqueness of each element's spectrum stems from the unique arrangement of its electrons in different energy levels. Each element has a specific number of protons in its nucleus, defining its atomic number and dictating the number of electrons it possesses in a neutral atom. This, in turn, determines the specific arrangement of electrons in energy levels and sublevels, leading to a unique set of energy differences between those levels.
Influence of Electron Configuration
The electron configuration, or the arrangement of electrons in the various shells and subshells, is crucial. For instance, hydrogen, with only one electron, has a much simpler energy level structure compared to iron, with 26 electrons occupying multiple shells and subshells. This complexity translates into a far more intricate emission spectrum for iron, exhibiting a greater number of spectral lines.
The Role of Subshells and Orbital Shapes
Even within a shell, electrons occupy different subshells (s, p, d, f), each characterized by a specific shape and energy. The interaction between electrons in these subshells adds to the complexity of the energy level structure, further contributing to the individuality of spectral lines. These subtle energy differences result in a rich and complex spectrum for each element.
Isotopes and Subtle Variations
While the fundamental spectrum is defined by the atomic number, isotopes of the same element (atoms with the same number of protons but different numbers of neutrons) may exhibit minute variations in their spectra due to subtle differences in nuclear mass and electron-nucleus interactions. These variations are typically insignificant compared to the overall uniqueness of the element's spectrum.
Applications of Atomic Spectra: Unraveling the Universe and Analyzing Matter
The unique spectral fingerprints of elements are invaluable tools across various scientific fields:
1. Astronomy: Unveiling the Composition of Stars and Galaxies
Astronomers utilize spectroscopy to analyze the light emitted from celestial objects. By identifying the spectral lines present in the light, they can determine the composition of stars, galaxies, and nebulae, providing crucial insights into their formation, evolution, and physical properties. This allows for the remote identification of elements across vast cosmic distances.
2. Analytical Chemistry: Identifying and Quantifying Elements
In analytical chemistry, atomic spectroscopy techniques like atomic absorption spectroscopy (AAS) and inductively coupled plasma optical emission spectroscopy (ICP-OES) are employed to identify and quantify elements in various samples, ranging from environmental pollutants to biological tissues. These methods leverage the unique spectral signatures to determine the elemental composition with high precision.
3. Forensic Science: Tracing Evidence and Identifying Substances
Forensic scientists also utilize spectral analysis to identify substances found at crime scenes. The unique spectral fingerprint of an element or compound can help link evidence to a suspect or establish the nature of unknown substances.
4. Material Science: Characterizing Materials
Material scientists use spectral analysis to characterize materials and determine their purity and composition. This technique plays a vital role in quality control and the development of new materials with desired properties.
Beyond Emission Spectra: Absorption Spectra
While emission spectra depict the light emitted by an element, absorption spectra demonstrate the light absorbed by an element. When light passes through a gas of a particular element, specific wavelengths corresponding to the energy differences between the element's electron energy levels are absorbed. This results in dark lines (absorption lines) in the spectrum at those specific wavelengths.
The absorption spectrum is essentially the inverse of the emission spectrum, providing another method for identifying elements. This principle is applied in various spectroscopic techniques and is crucial in astronomical observations, where astronomers analyze the light passing through interstellar gas clouds to determine their composition.
Conclusion: A Universe of Unique Signatures
The unique spectrum of each element is a direct consequence of the quantum nature of atoms and the quantized energy levels of electrons. The precise arrangement of electrons and the corresponding energy differences between levels lead to a unique set of emitted or absorbed photons, creating an unmistakable spectral fingerprint. This fundamental principle has profound implications for various scientific disciplines, allowing us to unravel the composition of distant stars, analyze terrestrial materials, and solve forensic mysteries. The universe, it turns out, is not only vast and beautiful but also incredibly well-organized, with each element possessing its own unique and identifiable spectral signature. The study of these spectra continues to expand our understanding of the cosmos and the matter that makes up our world.
Latest Posts
Latest Posts
-
The Simple Sugar Glucose Is An Example Of A
Apr 28, 2025
-
Which Components Of The Atom Have No Charge
Apr 28, 2025
-
How Many Electrons In The First Shell
Apr 28, 2025
-
A Monopolistically Competitive Firm Is One
Apr 28, 2025
-
Classify The Measurements As Having English Units Or Metric Units
Apr 28, 2025
Related Post
Thank you for visiting our website which covers about Why Does Each Element Have A Unique Spectrum . We hope the information provided has been useful to you. Feel free to contact us if you have any questions or need further assistance. See you next time and don't miss to bookmark.