Why Does Ionization Increase Across A Period
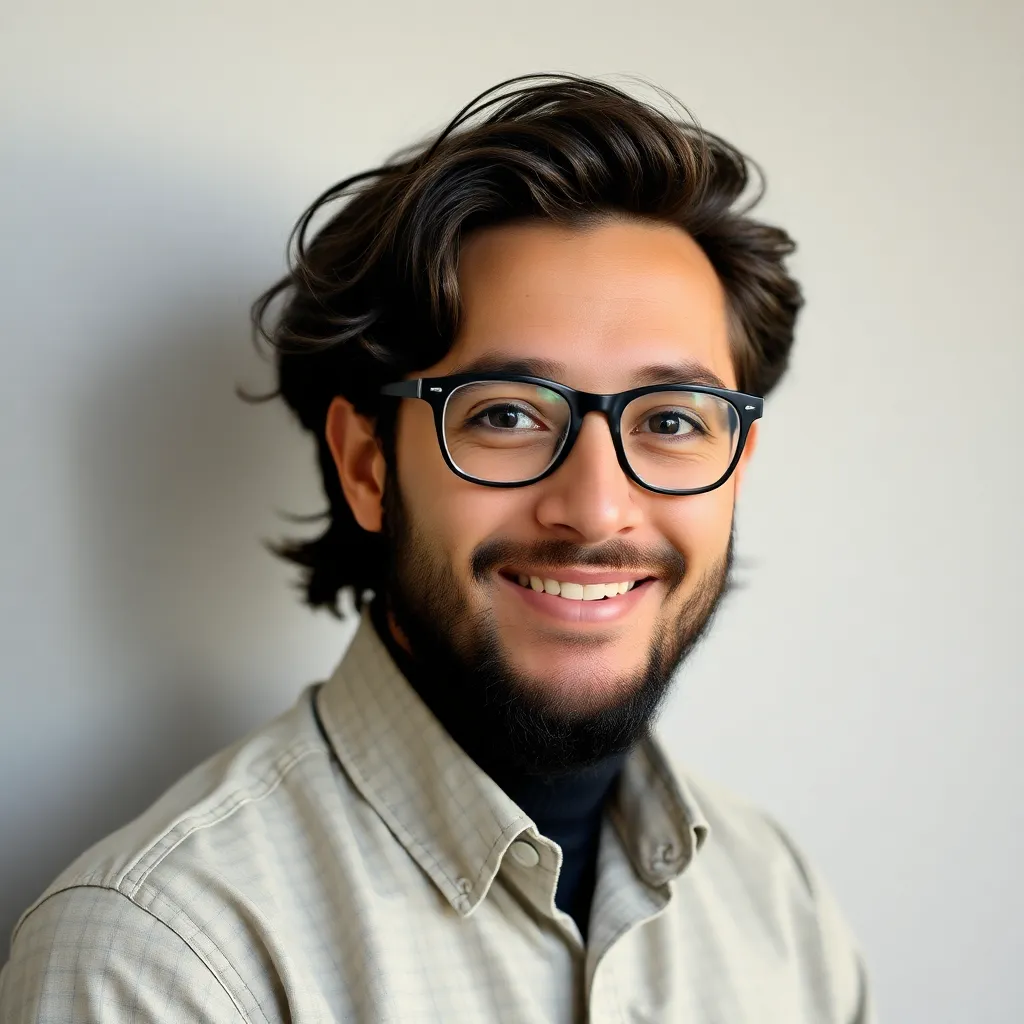
Muz Play
Apr 23, 2025 · 5 min read

Table of Contents
Why Does Ionization Energy Increase Across a Period? A Comprehensive Look
Ionization energy, the minimum energy required to remove an electron from a gaseous atom or ion, is a fundamental concept in chemistry. Understanding its trends across the periodic table is crucial for predicting the reactivity and properties of elements. This article delves deep into the reasons behind the increase in ionization energy across a period, exploring the underlying principles of atomic structure and electron configuration. We will also look at exceptions to this trend and the factors influencing ionization energy beyond the basic principles.
The Role of Effective Nuclear Charge
The primary reason for the increase in ionization energy across a period lies in the increasing effective nuclear charge. Effective nuclear charge (Z<sub>eff</sub>) represents the net positive charge experienced by an electron in a multi-electron atom. It's not simply the total number of protons in the nucleus (atomic number), but rather the charge felt after accounting for the shielding effect of other electrons.
Shielding and Penetration
Inner electrons shield outer electrons from the full positive charge of the nucleus. This shielding effect reduces the attractive force between the nucleus and the outer electrons. However, across a period, the number of protons increases while the electrons are added to the same principal energy level (shell). These added electrons are relatively poor at shielding each other from the increased nuclear charge.
Consequently, the effective nuclear charge experienced by the outermost electrons increases significantly across a period. This stronger pull from the nucleus makes it increasingly difficult to remove an electron, hence the increase in ionization energy. The stronger the pull, the more energy is required for ionization.
Illustrative Example: Sodium (Na) vs. Chlorine (Cl)
Let's compare sodium (Na), an alkali metal, and chlorine (Cl), a halogen, both in period 3. Sodium has 11 protons and 11 electrons, with one valence electron in the 3s orbital. Chlorine has 17 protons and 17 electrons, with seven valence electrons in the 3s and 3p orbitals.
While both elements have electrons in the same principal energy level (n=3), the chlorine nucleus possesses significantly more protons. The increased nuclear charge in chlorine, despite some shielding from inner electrons, results in a much stronger attraction for its valence electrons. This explains why chlorine has a considerably higher ionization energy than sodium.
Atomic Radius and Its Influence
Another factor contributing to the ionization energy trend is the atomic radius. As you move across a period, the atomic radius generally decreases. This decrease is a direct consequence of the increasing effective nuclear charge. The stronger pull from the nucleus draws the electrons closer, resulting in a smaller atomic size.
A smaller atomic radius implies that the outermost electrons are closer to the nucleus and experience a stronger attractive force. This proximity makes it more difficult to remove these electrons, further contributing to the higher ionization energy. The closer the electron is to the nucleus, the more energy is required to overcome the electrostatic attraction.
Electron-Electron Repulsion: A Minor Counteracting Force
While effective nuclear charge and atomic radius are dominant factors, electron-electron repulsion also plays a role, although it's generally less significant than the others. As more electrons are added to the same energy level across a period, they repel each other. This repulsion slightly reduces the effective nuclear charge felt by individual electrons.
However, this repulsive force is typically outweighed by the increase in effective nuclear charge, resulting in a net increase in ionization energy across a period. The increase in nuclear charge is simply more dominant than the increased electron-electron repulsion.
Exceptions to the General Trend
The general trend of increasing ionization energy across a period is not without exceptions. These exceptions arise primarily due to the stability associated with specific electron configurations.
Half-filled and Fully-filled Subshells
Elements with half-filled or fully-filled subshells exhibit slightly higher ionization energies than their neighbors. This increased stability arises from exchange energy and electron pairing energy.
-
Half-filled subshells: Electrons in a half-filled subshell (e.g., nitrogen with three electrons in the 2p subshell) experience less electron-electron repulsion because they occupy separate orbitals with parallel spins. This relatively lower repulsion leads to increased stability and a higher ionization energy.
-
Fully-filled subshells: Similar to half-filled subshells, fully filled subshells (e.g., neon with a complete 2p subshell) exhibit increased stability due to symmetrical electron distribution and minimal electron-electron repulsion. This enhances their ionization energy.
Beyond the Basics: A Deeper Dive
While the above explanations provide a good fundamental understanding, other subtleties influence ionization energy.
Penetration of Orbitals
Different orbitals within the same principal energy level have varying degrees of penetration, meaning they approach the nucleus to varying extents. Orbitals with higher penetration experience a stronger effective nuclear charge. For instance, in the 2p subshell, the electrons in the 2s orbital have a greater penetration and experience a higher effective nuclear charge than the electrons in the 2p orbital. This differential penetration slightly affects the observed ionization energy trend.
Relativistic Effects
For heavier elements, relativistic effects become significant. As electrons approach the nucleus at high speeds (particularly inner electrons), their mass increases due to relativistic effects. This increased mass causes the electrons to move closer to the nucleus and experience a stronger attraction. This effect further enhances ionization energy for higher atomic number elements.
Quantum Mechanical Considerations
A thorough understanding of ionization energy requires a quantum mechanical approach. The Schrödinger equation, or more advanced computational methods, must be employed to accurately determine the energy levels and wave functions of electrons. These calculations provide precise values for ionization energy, corroborating the trends observed experimentally.
Conclusion: A Complex interplay of Factors
The increase in ionization energy across a period is a consequence of a complex interplay of several factors, with effective nuclear charge and atomic radius being the most dominant. Shielding, electron-electron repulsion, orbital penetration, and relativistic effects (for heavier elements) contribute to subtle variations in the trend. Understanding these factors provides valuable insights into atomic behavior and the chemical properties of elements across the periodic table. The exceptions observed around half-filled and fully-filled subshells highlight the importance of electron configuration in determining atomic stability and ionization energy. A complete understanding requires incorporating quantum mechanical principles and appreciating the intricate interactions between electrons and the nucleus.
Latest Posts
Latest Posts
-
Which Body Cavity Affords The Least Protection
Apr 23, 2025
-
Chlorine 37 Can Be Represented As
Apr 23, 2025
-
What Are The Four Elements Found In All Proteins
Apr 23, 2025
-
Classify The Following Plants As Seed Or Seedless
Apr 23, 2025
-
An Epitope Associated With Which Part Of An Antibody
Apr 23, 2025
Related Post
Thank you for visiting our website which covers about Why Does Ionization Increase Across A Period . We hope the information provided has been useful to you. Feel free to contact us if you have any questions or need further assistance. See you next time and don't miss to bookmark.