3 Bond Pair 1 Lone Pair
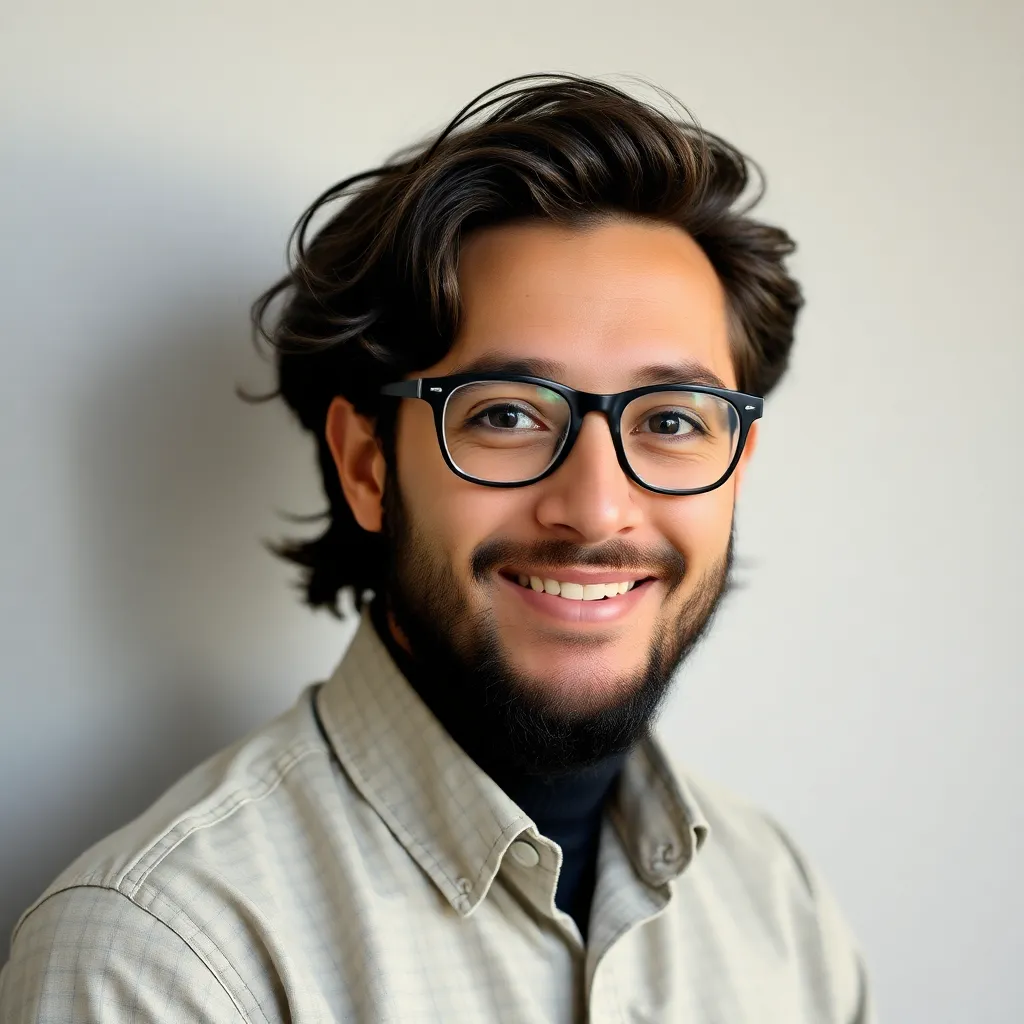
Muz Play
May 11, 2025 · 6 min read
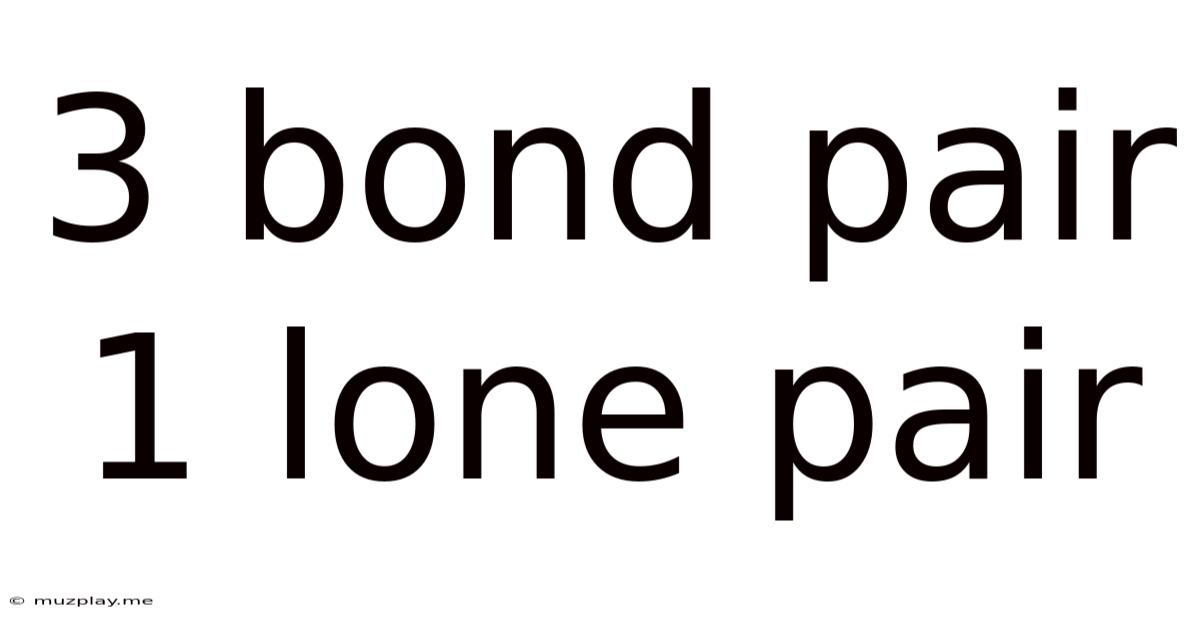
Table of Contents
3 Bond Pairs 1 Lone Pair: A Deep Dive into Molecular Geometry and Properties
Understanding molecular geometry is crucial in chemistry, as it dictates a molecule's physical and chemical properties. One common arrangement involves three bond pairs and one lone pair of electrons around a central atom. This configuration, often visualized using the Valence Shell Electron Pair Repulsion (VSEPR) theory, leads to a specific molecular geometry with unique characteristics. This article provides a comprehensive exploration of the 3 bond pair 1 lone pair arrangement, examining its geometry, bond angles, polarity, and examples, all while incorporating SEO best practices.
Understanding VSEPR Theory
Before delving into the specifics of a 3 bond pair 1 lone pair arrangement, it's essential to grasp the fundamental principles of VSEPR theory. This theory postulates that electron pairs – both bonding and non-bonding (lone pairs) – around a central atom repel each other and arrange themselves to minimize this repulsion. This arrangement determines the molecule's overall shape. The key is understanding that lone pairs exert a stronger repulsive force than bonding pairs, influencing the bond angles and molecular geometry.
Key VSEPR Concepts:
- Electron Domains: These represent regions of high electron density, encompassing both bonding pairs and lone pairs.
- Repulsion Strength: Lone pair-lone pair repulsion > lone pair-bond pair repulsion > bond pair-bond pair repulsion.
- Predicting Geometry: The number of electron domains predicts the electron domain geometry, while the number of lone pairs and bonding pairs determines the molecular geometry.
The Tetrahedral Electron Domain Geometry
A molecule with three bond pairs and one lone pair possesses a total of four electron domains. According to VSEPR theory, four electron domains arrange themselves in a tetrahedral geometry to maximize the distance between them. This is the electron domain geometry. The bond angles in a perfect tetrahedron are approximately 109.5 degrees. However, the presence of a lone pair significantly alters the molecular geometry.
Trigonal Pyramidal Molecular Geometry: The Impact of the Lone Pair
Because one of the electron domains is a lone pair, the resulting molecular geometry is not tetrahedral. The lone pair, occupying more space than a bonding pair, pushes the three bonding pairs closer together. This results in a trigonal pyramidal molecular geometry.
Visualizing the Trigonal Pyramid:
Imagine a pyramid with a triangular base. The central atom sits at the apex of the pyramid, with the three atoms bonded to it forming the vertices of the triangular base. The lone pair is positioned below the central atom, somewhat "hidden" but impacting the overall shape.
Bond Angles in Trigonal Pyramidal Structures:
While a perfect tetrahedron exhibits 109.5° bond angles, the presence of the lone pair compresses the bond angles in a trigonal pyramidal molecule. The bond angles are typically less than 109.5°, usually around 107°. This deviation stems from the stronger repulsive force exerted by the lone pair.
Polarity: A Consequence of Molecular Geometry
The molecular geometry of a molecule significantly impacts its polarity. A molecule is considered polar if it possesses a net dipole moment, meaning there's an uneven distribution of electron density. In a trigonal pyramidal molecule with three bond pairs and one lone pair, the molecule is generally polar, assuming the bonded atoms are not all identical.
Factors influencing Polarity:
- Electronegativity difference: The difference in electronegativity between the central atom and the bonded atoms contributes to the bond dipole moment.
- Molecular Geometry: The asymmetrical arrangement of atoms in a trigonal pyramidal structure prevents the bond dipoles from canceling each other out, resulting in a net dipole moment.
Examples of Molecules with 3 Bond Pairs and 1 Lone Pair
Many common molecules exhibit this arrangement. Understanding these examples solidifies the concept of the trigonal pyramidal geometry and its implications.
1. Ammonia (NH₃):
Ammonia is a classic example. The nitrogen atom (central atom) forms three covalent bonds with three hydrogen atoms, and possesses one lone pair of electrons. This leads to the characteristic trigonal pyramidal structure and makes ammonia a polar molecule.
2. Phosphine (PH₃):
Similar to ammonia, phosphine also exhibits a trigonal pyramidal geometry. The phosphorus atom is the central atom, bonded to three hydrogen atoms with one lone pair. However, the P-H bond is less polar than the N-H bond due to the lower electronegativity of phosphorus compared to nitrogen.
3. Nitrogen Trifluoride (NF₃):
Nitrogen trifluoride shares the same electron domain and molecular geometry as ammonia and phosphine. The nitrogen atom is bonded to three fluorine atoms, and possesses one lone pair. Despite the electronegativity difference between nitrogen and fluorine, the molecule's overall polarity is surprisingly low compared to expectations. This highlights the complexities of predicting polarity based solely on electronegativity differences. The lone pair plays a significant role in the overall dipole moment.
4. Arsenic Trifluoride (AsF₃):
Arsenic trifluoride is analogous to nitrogen trifluoride, exhibiting a trigonal pyramidal structure.
Comparing Trigonal Pyramidal to Other Geometries
Understanding the differences between trigonal pyramidal and other geometries helps solidify this concept within the broader context of VSEPR theory.
Trigonal Pyramidal vs. Tetrahedral:
The key distinction lies in the presence of a lone pair. A tetrahedral molecule has four bonding pairs, resulting in a symmetrical structure. In contrast, the lone pair in a trigonal pyramidal molecule disrupts the symmetry, leading to the pyramidal shape.
Trigonal Pyramidal vs. Trigonal Planar:
A trigonal planar molecule has three bonding pairs and zero lone pairs. This leads to a flat, triangular structure with bond angles of 120°. The lone pair in a trigonal pyramidal molecule pushes the bonding pairs closer, reducing the bond angles and creating a three-dimensional structure.
Applications and Importance
Understanding the 3 bond pair 1 lone pair arrangement has significant applications across various fields:
- Predicting Molecular Properties: Knowledge of geometry is vital for predicting properties like polarity, reactivity, and boiling points.
- Drug Design: Molecular shape is crucial in drug design, as it influences how a drug interacts with its target receptor.
- Material Science: Understanding molecular geometry helps in designing materials with specific properties.
- Catalysis: The shape and polarity of molecules play crucial roles in catalytic reactions.
Conclusion
The 3 bond pair 1 lone pair arrangement, resulting in a trigonal pyramidal molecular geometry, is a fundamental concept in chemistry. This article explored the underlying principles of VSEPR theory, the impact of lone pairs on bond angles and molecular polarity, and provided several examples of common molecules exhibiting this arrangement. Understanding this geometry is crucial for predicting and interpreting the properties of many important compounds, underscoring its importance across various scientific disciplines. Further exploration of advanced concepts like hybridization and molecular orbital theory can provide an even deeper understanding of the intricacies of this significant molecular configuration.
Latest Posts
Latest Posts
-
How To Do Bohr Rutherford Diagrams
May 12, 2025
-
Is Milk Pure Substance Or Mixture
May 12, 2025
-
Power Series Of 1 1 X
May 12, 2025
-
Is Boron Trifluoride Polar Or Nonpolar
May 12, 2025
-
Which Point Of The Beam Experiences The Most Compression
May 12, 2025
Related Post
Thank you for visiting our website which covers about 3 Bond Pair 1 Lone Pair . We hope the information provided has been useful to you. Feel free to contact us if you have any questions or need further assistance. See you next time and don't miss to bookmark.