After Glycolysis The Pyruvate Molecules Go To The
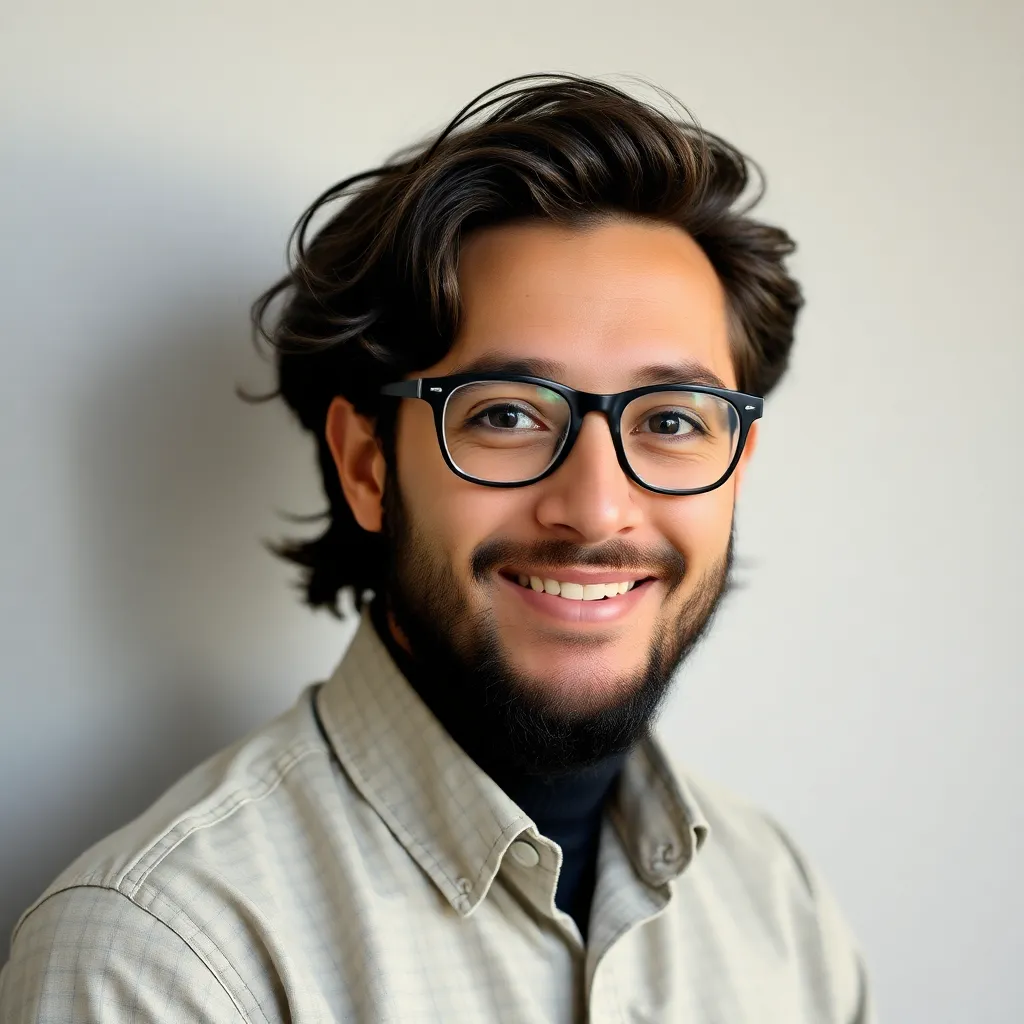
Muz Play
Apr 05, 2025 · 6 min read
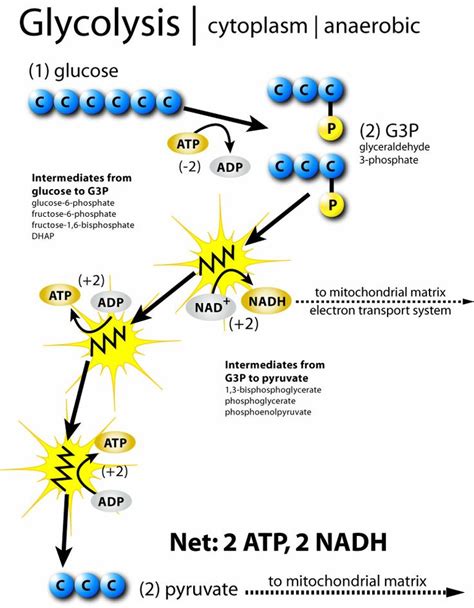
Table of Contents
After Glycolysis: The Fate of Pyruvate Molecules
Glycolysis, the metabolic pathway that breaks down glucose into pyruvate, is a crucial initial step in cellular respiration. But the journey of energy production doesn't end there. The fate of those pyruvate molecules, generated in the cytoplasm, depends critically on the presence or absence of oxygen. This branching point dictates whether cellular respiration proceeds through aerobic or anaerobic pathways, profoundly impacting the overall energy yield and the byproducts produced. This article delves into the intricacies of pyruvate's post-glycolytic journey, exploring both aerobic and anaerobic fates and highlighting the critical regulatory mechanisms involved.
The Aerobic Pathway: Pyruvate's Journey to the Mitochondria
In the presence of oxygen, pyruvate molecules embark on a remarkable journey into the mitochondria, the powerhouse of the cell. This aerobic pathway is far more efficient in terms of ATP (adenosine triphosphate) production than its anaerobic counterpart. This journey involves several key steps:
1. Transport Across the Mitochondrial Membrane
Pyruvate, a charged molecule, cannot passively diffuse across the mitochondrial membrane. Instead, it relies on a specific transporter protein, the pyruvate transporter, which facilitates its active transport into the mitochondrial matrix. This transport is a crucial step, ensuring that pyruvate reaches the site of the next metabolic stage: the pyruvate dehydrogenase complex (PDC) reaction. The efficiency of this transport directly influences the rate of subsequent aerobic respiration.
2. The Pyruvate Dehydrogenase Complex (PDC) Reaction: Decarboxylation and Acetyl-CoA Formation
Once inside the mitochondrial matrix, pyruvate encounters the PDC, a multi-enzyme complex responsible for converting pyruvate into acetyl-CoA. This pivotal reaction involves several key steps:
-
Decarboxylation: A carboxyl group (-COOH) is removed from pyruvate, releasing carbon dioxide (CO2) as a byproduct. This is an irreversible step, committing the pyruvate molecule to the aerobic pathway.
-
Oxidation: Pyruvate undergoes oxidation, losing electrons. These electrons are transferred to NAD+, reducing it to NADH. NADH is a crucial electron carrier, playing a vital role in the electron transport chain (ETC) later in the process.
-
Coenzyme A (CoA) Attachment: The remaining two-carbon acetyl group is attached to Coenzyme A (CoA), forming acetyl-CoA. Acetyl-CoA is a pivotal molecule, representing the entry point for the citric acid cycle (also known as the Krebs cycle or TCA cycle).
The activity of the PDC is tightly regulated to ensure a balanced energy production. Several factors influence its activity, including the levels of its substrates (pyruvate and CoA), products (acetyl-CoA and NADH), and allosteric inhibitors and activators. For instance, high levels of NADH and acetyl-CoA inhibit PDC activity, preventing the overproduction of acetyl-CoA when the citric acid cycle is already operating at full capacity.
3. The Citric Acid Cycle: Energy Extraction from Acetyl-CoA
Acetyl-CoA, the product of the PDC reaction, enters the citric acid cycle, a series of eight enzymatic reactions that further oxidize the acetyl group, extracting energy in the form of ATP, NADH, and FADH2 (another electron carrier). Each turn of the cycle generates:
- 1 ATP (or GTP): Via substrate-level phosphorylation.
- 3 NADH: Carrying high-energy electrons to the ETC.
- 1 FADH2: Carrying high-energy electrons to the ETC.
- 2 CO2: Released as a byproduct.
The citric acid cycle is a central metabolic hub, connecting various metabolic pathways. Its intermediates are not only involved in energy production but also serve as precursors for the biosynthesis of amino acids, fatty acids, and other essential molecules. The regulation of the citric acid cycle ensures that it operates efficiently and responds appropriately to cellular energy demands.
4. Oxidative Phosphorylation: The Electron Transport Chain and Chemiosmosis
The NADH and FADH2 generated during the PDC reaction and the citric acid cycle carry high-energy electrons to the electron transport chain (ETC), located in the inner mitochondrial membrane. The ETC consists of a series of protein complexes that transfer electrons from NADH and FADH2 down an energy gradient. This electron flow drives the pumping of protons (H+) across the inner mitochondrial membrane, creating a proton gradient.
This proton gradient represents stored potential energy. The energy is then harnessed through chemiosmosis, where protons flow back across the inner membrane through ATP synthase, an enzyme that catalyzes the synthesis of ATP from ADP and inorganic phosphate (Pi). This process, oxidative phosphorylation, is responsible for the vast majority of ATP production during aerobic respiration. A single glucose molecule, through complete aerobic respiration, can yield up to 36-38 ATP molecules.
The Anaerobic Pathway: Fermentation
In the absence of oxygen, pyruvate's fate diverges significantly. Anaerobic respiration, also known as fermentation, is a less efficient pathway, yielding a much smaller ATP harvest. Two primary types of fermentation are common:
1. Lactic Acid Fermentation
In lactic acid fermentation, pyruvate is directly reduced by NADH to produce lactic acid. This reaction regenerates NAD+, which is crucial for maintaining glycolysis. The process is relatively simple, but the energy yield is meager, producing only 2 ATP molecules per glucose molecule (the net yield from glycolysis itself). Lactic acid fermentation is common in muscle cells during intense exercise when oxygen supply is limited, and it's also used by certain bacteria in the production of yogurt and other fermented foods. The accumulation of lactic acid can lead to muscle fatigue and soreness.
2. Alcoholic Fermentation
In alcoholic fermentation, pyruvate is first decarboxylated to acetaldehyde, releasing CO2. Then, acetaldehyde is reduced by NADH to produce ethanol. Similar to lactic acid fermentation, this process regenerates NAD+, allowing glycolysis to continue. Alcoholic fermentation is employed by yeast and some bacteria in the production of alcoholic beverages and bread. The CO2 produced during alcoholic fermentation is responsible for the rise of bread dough.
Regulation of Pyruvate Metabolism: A Complex Interplay
The metabolic fate of pyruvate is tightly regulated, ensuring that the cell responds appropriately to changing energy demands and oxygen availability. Several key regulatory mechanisms are involved:
-
Oxygen Availability: The presence or absence of oxygen is the primary determinant of pyruvate's metabolic pathway. High oxygen levels promote aerobic respiration, while low oxygen levels trigger anaerobic fermentation.
-
Enzyme Activity: The activity of key enzymes involved in pyruvate metabolism, such as the PDC and lactate dehydrogenase (LDH) in lactic acid fermentation, are regulated by allosteric modulation and covalent modification. These mechanisms ensure that the metabolic pathways are finely tuned to meet the cell's energy needs.
-
Energy Charge: The cellular energy charge, reflecting the ratio of ATP to ADP, influences the activity of enzymes involved in pyruvate metabolism. High energy charge inhibits further ATP production, while low energy charge stimulates metabolic pathways to generate more ATP.
-
Hormonal Control: Hormones, such as insulin and glucagon, play a role in regulating glucose metabolism and consequently influence the metabolic fate of pyruvate.
Conclusion: A Crucial Metabolic Crossroads
The post-glycolytic fate of pyruvate represents a critical metabolic crossroads, determining the efficiency of energy production and the types of byproducts generated. The choice between aerobic and anaerobic pathways is governed primarily by oxygen availability, but other factors, such as energy charge and hormonal regulation, play important roles. Understanding the intricacies of pyruvate metabolism is crucial for comprehending cellular respiration, energy production, and various metabolic disorders. Further research into the regulatory mechanisms and the interplay between various metabolic pathways will continue to enhance our understanding of this fundamental biological process. The study of pyruvate metabolism highlights the cell's remarkable ability to adapt and optimize its energy production strategies in response to changing environmental conditions.
Latest Posts
Latest Posts
-
Bartolome De Las Casas Describes The Exploitation Of Indigenous Peoples
Apr 06, 2025
-
The Structure Labeled A On The Transcription Diagram Is
Apr 06, 2025
-
Difference Between Atomic Mass And Molar Mass
Apr 06, 2025
-
What Does It Mean If A Reaction Is Spontaneous
Apr 06, 2025
-
According To Mendels Law Of Independent Assortment
Apr 06, 2025
Related Post
Thank you for visiting our website which covers about After Glycolysis The Pyruvate Molecules Go To The . We hope the information provided has been useful to you. Feel free to contact us if you have any questions or need further assistance. See you next time and don't miss to bookmark.