Amino Acid Charges At Physiological Ph
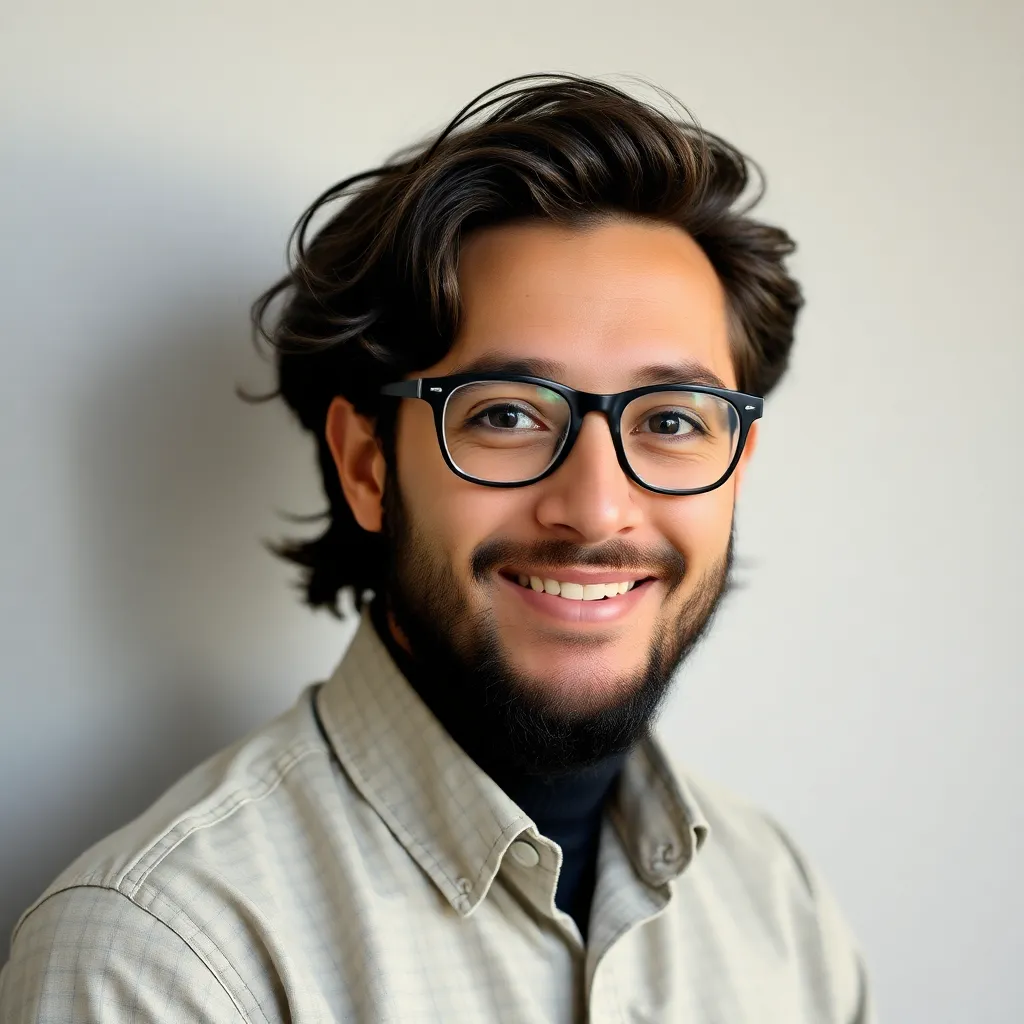
Muz Play
Apr 08, 2025 · 7 min read

Table of Contents
Amino Acid Charges at Physiological pH: A Comprehensive Guide
Understanding the charge of amino acids at physiological pH (around 7.4) is crucial for comprehending protein structure, function, and interactions. This property significantly influences protein folding, enzyme activity, and interactions with other molecules within the biological system. This detailed guide will explore the intricacies of amino acid charges at physiological pH, covering their classification, pKa values, titration curves, and the implications of their charge for biological processes.
The Importance of Amino Acid Charge in Biological Systems
Proteins, the workhorses of the cell, are polymers composed of amino acids linked together by peptide bonds. The unique sequence of amino acids, along with their individual properties, dictates the overall protein structure and, consequently, its function. One of the most critical properties of amino acids is their charge at a given pH. At physiological pH, the charge of each amino acid side chain (R-group) contributes significantly to the overall charge of the protein. This net charge affects many aspects of protein behavior including:
-
Protein Folding and Stability: The interactions between charged amino acid residues are essential for maintaining the correct three-dimensional structure of a protein. Electrostatic interactions, such as salt bridges (between oppositely charged residues), significantly contribute to the stability of the folded protein.
-
Enzyme Activity: The active site of an enzyme, the region where substrate binding and catalysis occur, often relies on precisely positioned charged amino acid residues to attract, bind, and orient substrates for optimal reaction rates. Changes in pH, altering the charge of these residues, can significantly impact enzyme activity.
-
Protein-Protein Interactions: Many biological processes depend on specific interactions between proteins. Electrostatic interactions between oppositely charged regions on different proteins play a crucial role in these interactions, contributing to signaling pathways, immune responses, and many other vital cellular functions.
-
Protein-Ligand Interactions: Similarly, the binding of proteins to ligands (small molecules, ions, etc.) often involves electrostatic interactions, driven by the charge distribution on both the protein and the ligand. This is critical for processes like receptor-ligand binding, transport, and signal transduction.
Amino Acid Classification Based on Side Chain Charge at Physiological pH
Amino acids are typically categorized into several groups based on the properties of their side chains, including their charge at physiological pH:
-
Nonpolar, Aliphatic Amino Acids: These amino acids possess hydrophobic side chains with no significant charge at physiological pH. Examples include Glycine (Gly, G), Alanine (Ala, A), Valine (Val, V), Leucine (Leu, L), Isoleucine (Ile, I), Methionine (Met, M), and Proline (Pro, P).
-
Aromatic Amino Acids: These amino acids have aromatic side chains. While some may exhibit weak polarity, they generally carry no net charge at physiological pH. Examples include Phenylalanine (Phe, F), Tyrosine (Tyr, Y), and Tryptophan (Trp, W).
-
Polar, Uncharged Amino Acids: These amino acids possess polar side chains capable of forming hydrogen bonds but carry no net charge at physiological pH. Examples include Serine (Ser, S), Threonine (Thr, T), Cysteine (Cys, C), Asparagine (Asn, N), and Glutamine (Gln, Q).
-
Positively Charged (Basic) Amino Acids: These amino acids have positively charged side chains at physiological pH. They are crucial for ionic interactions within and between proteins. Examples include Lysine (Lys, K), Arginine (Arg, R), and Histidine (His, H). Histidine is unique due to its pKa being close to physiological pH, meaning its charge can be significantly influenced by small pH changes.
-
Negatively Charged (Acidic) Amino Acids: These amino acids have negatively charged side chains (carboxylate groups) at physiological pH. These negatively charged residues play a vital role in electrostatic interactions and contribute significantly to the overall protein charge. Examples include Aspartic Acid (Asp, D) and Glutamic Acid (Glu, E).
Understanding pKa Values and Titration Curves
The charge of an amino acid's side chain at a specific pH is determined by its pKa value. The pKa is the pH at which half of the molecules of a given species are protonated and half are deprotonated. Each ionizable group on an amino acid (carboxyl group, amino group, and side chain if ionizable) has its own pKa value.
A titration curve illustrates the relationship between pH and the degree of ionization of an amino acid. By observing the titration curve, one can determine the pKa values of the ionizable groups and predict the charge of the amino acid at any given pH. At pH values significantly below the pKa of an ionizable group, the group will be predominantly protonated (positively charged or neutral). At pH values significantly above the pKa, the group will be predominantly deprotonated (negatively charged or neutral). Near the pKa, a significant fraction of molecules will exist in both protonated and deprotonated forms.
Example: Glutamic Acid
Glutamic acid has three ionizable groups: an α-carboxyl group (pKa ~2.2), an α-amino group (pKa ~9.7), and a side-chain carboxyl group (pKa ~4.3).
- At pH 7.4 (physiological pH): The α-carboxyl group and the side-chain carboxyl group will be predominantly deprotonated (negatively charged), while the α-amino group will be predominantly protonated (positively charged). This results in a net negative charge on the glutamic acid molecule at physiological pH.
The Unique Case of Histidine
Histidine's imidazole side chain has a pKa value of approximately 6.0, making it unique among the amino acids. This pKa is close to physiological pH (7.4), meaning that histidine can exist in both protonated (positively charged) and deprotonated (neutral) forms at physiological pH. This property allows histidine to act as a pH buffer and play a crucial role in many enzyme active sites, facilitating catalysis by accepting or donating protons depending on the reaction conditions.
The proportion of protonated and deprotonated histidine at physiological pH depends on the microenvironment of the histidine residue within the protein. Factors such as nearby charged residues, hydrogen bonding networks, and the overall protein structure can significantly influence the effective pKa of histidine, shifting it away from its typical value.
Implications for Protein Structure and Function
The overall charge of a protein is the sum of the charges of its constituent amino acids at a given pH. This net charge significantly impacts protein behavior:
-
Solubility: Proteins with a high net charge are generally more soluble in aqueous solutions due to increased interactions with water molecules.
-
Interactions with other molecules: Electrostatic interactions between charged residues on a protein and other molecules (e.g., DNA, other proteins, ligands) play a critical role in various biological processes.
-
Protein Stability: Salt bridges between oppositely charged residues contribute significantly to protein stability. Disrupting these interactions, for instance by altering the pH, can lead to protein unfolding (denaturation).
-
Enzyme Mechanism: Many enzymes rely on precisely positioned charged residues within their active sites to bind substrates and catalyze reactions.
Factors Affecting Amino Acid Charge at Physiological pH
Several factors beyond the inherent pKa values of amino acids can influence their charge at physiological pH:
-
Microenvironment: The local environment within a protein significantly impacts the pKa of ionizable side chains. The presence of charged residues nearby, hydrogen bonding interactions, and hydrophobic environments can alter the effective pKa, shifting it higher or lower than the standard value.
-
pH variations: While physiological pH is relatively stable, localized pH changes within cellular compartments can significantly affect the charge of amino acid residues, influencing protein function and interactions.
-
Post-translational modifications: Certain post-translational modifications, such as phosphorylation (addition of a phosphate group), can introduce new charges to amino acid side chains, altering the overall protein charge and function.
Applications and Further Research
Understanding amino acid charges at physiological pH is crucial in various fields:
-
Drug Design: Understanding the electrostatic interactions between drugs and their target proteins is essential for designing effective pharmaceuticals.
-
Protein Engineering: Modifying the charge distribution of a protein can alter its properties and functions, making it a powerful tool in biotechnology.
-
Proteomics: Studying the overall charge distribution of proteins within biological systems provides valuable insights into cellular processes.
Further research continues to explore the intricate details of amino acid charges and their impact on protein structure, function, and interactions. Advanced computational techniques and experimental methods are constantly refining our understanding of this fundamental aspect of biochemistry. Continued advancements in this field will undoubtedly lead to breakthroughs in various biomedical and biotechnological applications.
Latest Posts
Latest Posts
-
Using Nonsense Syllables To Study Memory Hermann Ebbinghaus Found That
Apr 18, 2025
-
Determine The Oxidation Number Of Each Element In If
Apr 18, 2025
-
Every Chemical Reaction Involves A Change In
Apr 18, 2025
-
How Do You Clear An Equation Of Fractions
Apr 18, 2025
-
1 125 Rounded To The Nearest Tenth
Apr 18, 2025
Related Post
Thank you for visiting our website which covers about Amino Acid Charges At Physiological Ph . We hope the information provided has been useful to you. Feel free to contact us if you have any questions or need further assistance. See you next time and don't miss to bookmark.