Continuous Propagation Of The Action Potential
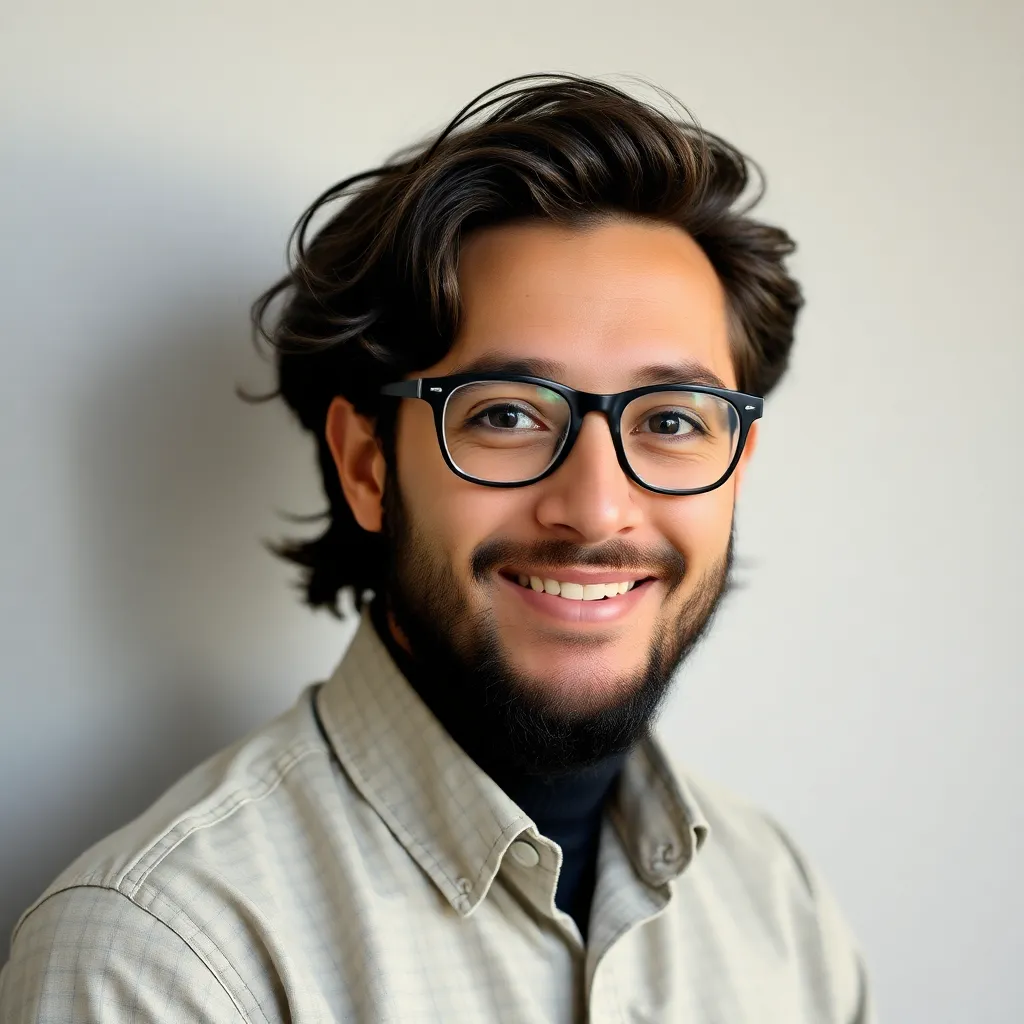
Muz Play
May 12, 2025 · 6 min read
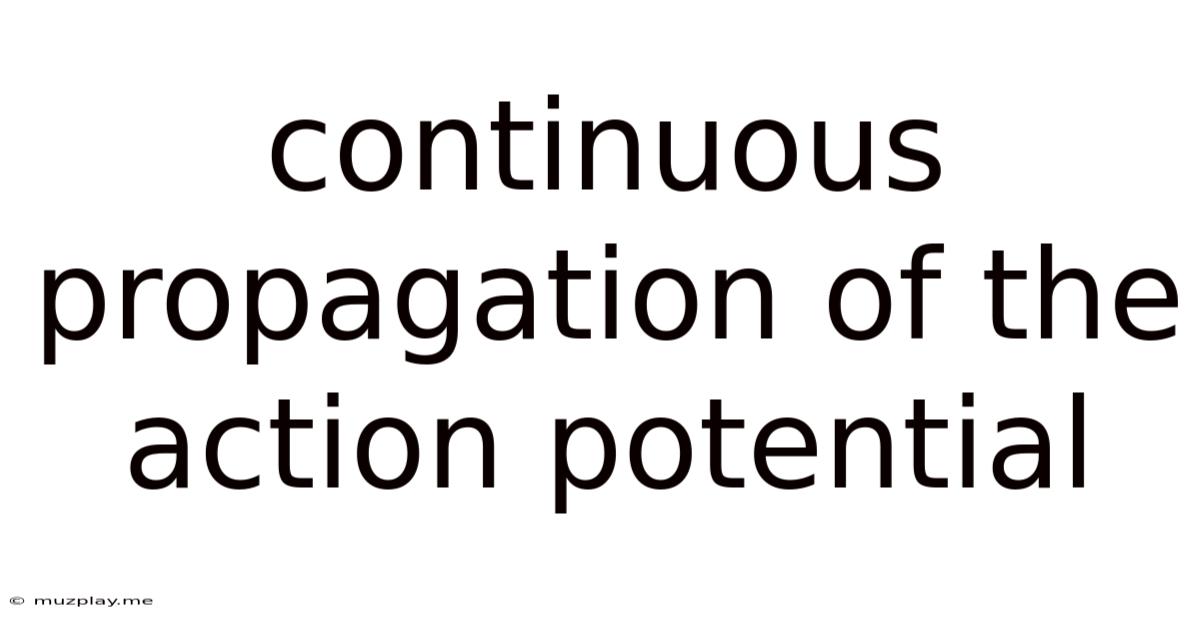
Table of Contents
Continuous Propagation of the Action Potential: A Deep Dive
The propagation of the action potential, the electrical signal that allows neurons to communicate, is a fundamental process in neurobiology. Understanding how this signal travels down the axon – the long, slender projection of a neuron – is crucial to comprehending the intricacies of the nervous system. While some axons employ saltatory conduction, a faster method involving myelinated segments, many others rely on continuous propagation. This article delves into the detailed mechanisms of continuous propagation, exploring the ionic currents, refractory periods, and factors influencing conduction velocity.
Understanding the Action Potential
Before diving into continuous propagation, let's briefly review the action potential itself. This is a rapid, transient depolarization of the neuronal membrane, caused by the influx of sodium ions (Na⁺) and followed by repolarization due to the efflux of potassium ions (K⁺). This all-or-nothing event occurs when the membrane potential reaches a critical threshold, triggering a cascade of events.
The Key Players: Voltage-Gated Ion Channels
The action potential is orchestrated by voltage-gated ion channels embedded within the neuronal membrane. These channels are exquisitely sensitive to changes in membrane potential.
- Voltage-gated Sodium Channels (Naᵥ): These channels open rapidly upon depolarization, allowing a large influx of Na⁺ into the cell, causing further depolarization. This positive feedback loop is crucial for the rapid rising phase of the action potential.
- Voltage-gated Potassium Channels (Kᵥ): These channels open more slowly than Naᵥ channels. Their opening allows K⁺ to flow out of the cell, repolarizing the membrane and returning it to its resting potential.
The Stages of an Action Potential
The action potential proceeds through several distinct phases:
- Resting Potential: The membrane is polarized, typically around -70 mV.
- Depolarization: A stimulus causes the membrane potential to reach the threshold, triggering the opening of Naᵥ channels. Na⁺ influx rapidly depolarizes the membrane.
- Overshoot: The membrane potential becomes positive, peaking at around +30 mV.
- Repolarization: Naᵥ channels inactivate, and Kᵥ channels open, allowing K⁺ efflux and repolarizing the membrane.
- Hyperpolarization: Kᵥ channels remain open slightly longer than necessary, causing a brief hyperpolarization below the resting potential.
- Return to Resting Potential: The membrane potential gradually returns to its resting state.
Continuous Propagation: A Step-by-Step Explanation
Continuous propagation, unlike saltatory conduction, involves the sequential depolarization of adjacent membrane regions along the entire length of the unmyelinated axon. The process unfolds as follows:
- Initial Depolarization: An action potential is initiated at the axon hillock (the region where the axon originates from the soma). This initial depolarization is caused by a sufficient summation of excitatory postsynaptic potentials (EPSPs).
- Local Current Flow: The influx of Na⁺ during depolarization at one point creates a local current flow. This current spreads passively along the axon, depolarizing adjacent membrane regions.
- Threshold Exceeded: If the depolarization in the adjacent region reaches the threshold potential, it triggers the opening of Naᵥ channels in that region, initiating a new action potential.
- Sequential Depolarization: This process repeats itself along the length of the axon, with each action potential triggering the next in a sequential manner. The action potential effectively "travels" down the axon.
- Refractory Period: The refractory period, a period following an action potential during which another action potential cannot be initiated, ensures unidirectional propagation. This is due to the inactivation of Naᵥ channels and the continued outflow of K⁺.
Factors Influencing Conduction Velocity in Continuous Propagation
Several factors influence how quickly the action potential travels down the axon during continuous propagation:
- Axon Diameter: Larger diameter axons have lower internal resistance, allowing for faster spread of the local current and therefore a faster conduction velocity. Think of it like a wider pipe allowing water to flow more easily.
- Membrane Resistance: A higher membrane resistance reduces leakage of current across the membrane, leading to a faster conduction velocity. A less leaky membrane is more efficient in propagating the signal.
- Membrane Capacitance: Lower membrane capacitance means less charge needs to be accumulated to depolarize the membrane to the threshold, resulting in faster conduction. A lower capacitance is like a smaller capacitor that charges up quicker.
- Temperature: Higher temperatures generally increase the rate of ion channel opening and closing, leading to faster conduction velocity. Conversely, lower temperatures slow conduction.
The Role of the Refractory Period
The refractory period plays a critical role in the unidirectional propagation of the action potential. This period is divided into two phases:
- Absolute Refractory Period: During this period, no stimulus, no matter how strong, can elicit another action potential. This is due to the inactivation of Naᵥ channels.
- Relative Refractory Period: During this period, a stronger-than-normal stimulus can elicit an action potential. This is because some Naᵥ channels have recovered from inactivation, but the membrane is still hyperpolarized due to the continued outflow of K⁺.
The refractory period ensures that the action potential propagates in one direction only, preventing it from traveling backward. This is crucial for efficient and reliable neuronal signaling.
Continuous vs. Saltatory Conduction: A Comparison
While continuous propagation is found in unmyelinated axons, saltatory conduction occurs in myelinated axons. The key difference lies in the way the action potential travels:
Feature | Continuous Propagation | Saltatory Conduction |
---|---|---|
Myelin Sheath | Absent | Present |
Propagation | Sequential depolarization of adjacent membrane regions | Depolarization at Nodes of Ranvier |
Conduction Velocity | Relatively slow | Relatively fast |
Energy Consumption | Higher | Lower |
Axon Type | Unmyelinated axons | Myelinated axons |
Saltatory conduction, due to its faster speed and lower energy requirement, is a more efficient method of propagation. However, continuous propagation serves its purpose in various neuronal circuits.
Clinical Implications and Research
Dysfunctions in the mechanisms underlying continuous propagation can have significant clinical implications. Various neurological disorders can affect axon function, potentially leading to impaired neuronal communication. Research into the specifics of continuous propagation is ongoing and critical for understanding these conditions and developing effective treatments. For example, studies exploring the effects of various toxins and diseases on ion channel function are constantly expanding our understanding of neuronal signaling.
Further research into the interplay of the various factors affecting conduction velocity, especially in different types of neurons, promises to provide a more complete picture of neuronal communication and its role in health and disease. This includes investigating the role of different ion channel subtypes and their modulation by various factors.
Conclusion
Continuous propagation of the action potential is a fundamental process enabling neuronal communication in unmyelinated axons. The intricate interplay of voltage-gated ion channels, membrane properties, and the refractory period ensures the unidirectional and reliable transmission of the electrical signal. Understanding the factors influencing conduction velocity is vital for comprehending the complexities of the nervous system and exploring potential therapeutic interventions for neurological disorders. Further research is needed to fully elucidate the nuances of this vital process and its implications for human health. The continuous advancements in neurobiology continue to shed light on this remarkable aspect of neuronal function, constantly refining our understanding of how the brain and nervous system operate. The study of continuous propagation not only enhances our understanding of basic neurophysiology but also offers crucial insights into a variety of neurological conditions. Continued research in this field will undoubtedly contribute significantly to advancements in neuroscience and medicine.
Latest Posts
Latest Posts
-
Write An Equation For The Quadratic Graphed Below
May 12, 2025
-
Give The Solution Set To The System Of Equations
May 12, 2025
-
What Happens To An Animal Cell In A Hypotonic Solution
May 12, 2025
-
Atomic Radius Of Hydrogen In Pm
May 12, 2025
-
Effective Nuclear Charge And Ionization Energy
May 12, 2025
Related Post
Thank you for visiting our website which covers about Continuous Propagation Of The Action Potential . We hope the information provided has been useful to you. Feel free to contact us if you have any questions or need further assistance. See you next time and don't miss to bookmark.