Effective Nuclear Charge And Ionization Energy
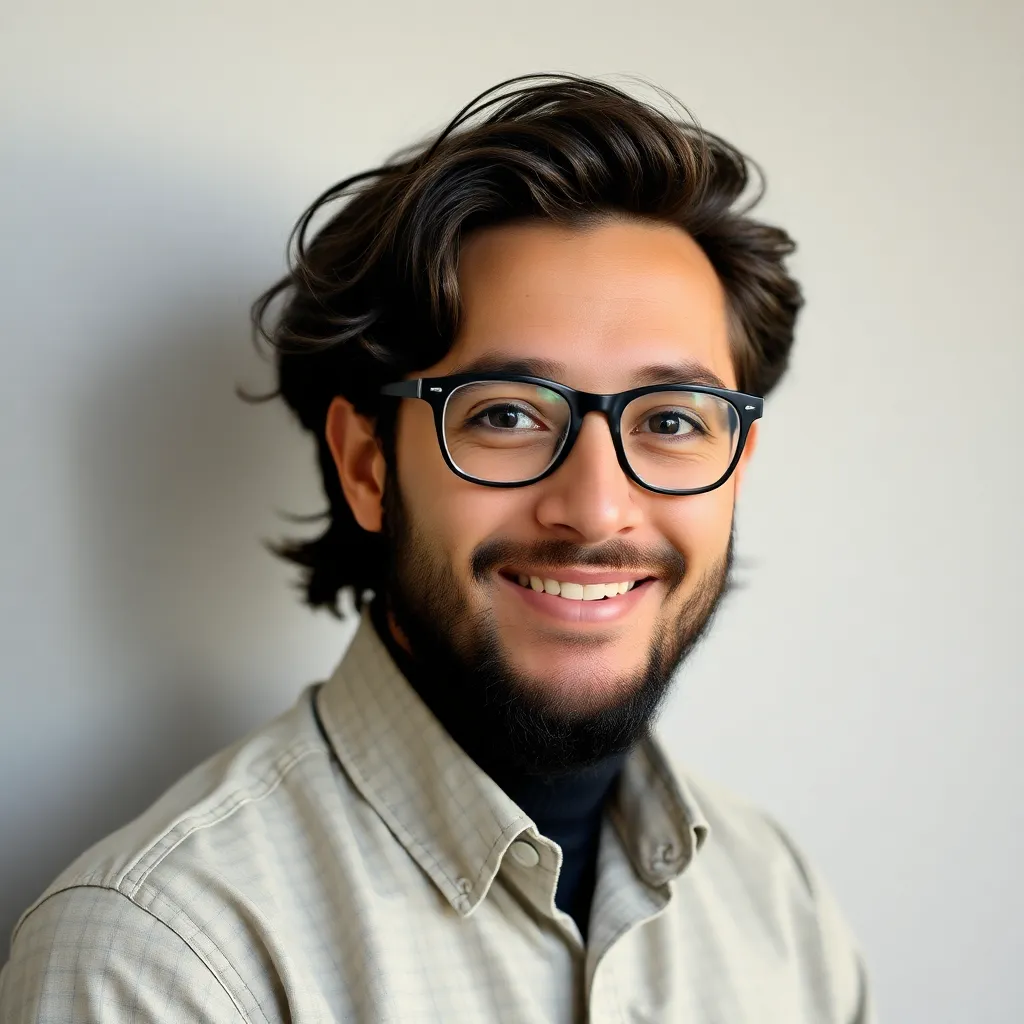
Muz Play
May 12, 2025 · 7 min read
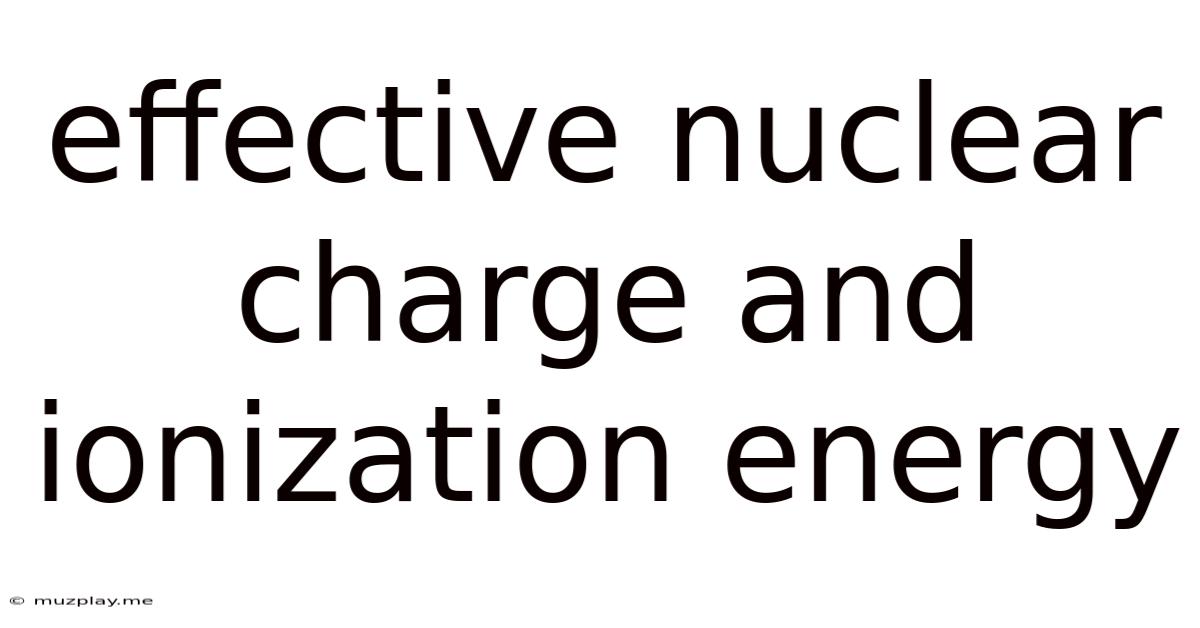
Table of Contents
Effective Nuclear Charge and Ionization Energy: A Deep Dive
Understanding the behavior of atoms and their interactions is fundamental to chemistry. Two key concepts that illuminate this behavior are effective nuclear charge (Z<sub>eff</sub>) and ionization energy (IE). While seemingly distinct, these properties are intrinsically linked, with effective nuclear charge significantly influencing the magnitude of ionization energy. This article explores both concepts in detail, explaining their definitions, calculating methods, periodic trends, and the crucial relationship between them.
What is Effective Nuclear Charge (Z<sub>eff</sub>)?
The effective nuclear charge represents the net positive charge experienced by an electron in a multi-electron atom. It's not simply the total positive charge of the nucleus (the atomic number, Z), because inner electrons shield outer electrons from the full attractive force of the protons. Imagine the nucleus as a powerful magnet, and the inner electrons as a layer of weaker magnets positioned between the nucleus and outer electrons. These inner electrons partially "block" or "shield" the outer electrons from the full pull of the nucleus.
Therefore, Z<sub>eff</sub> is always less than Z. The difference between Z and Z<sub>eff</sub> reflects the shielding effect. A higher Z<sub>eff</sub> means a stronger attraction between the nucleus and the outer electrons, while a lower Z<sub>eff</sub> signifies a weaker attraction.
Calculating Effective Nuclear Charge
Precisely calculating Z<sub>eff</sub> is complex, requiring advanced quantum mechanical methods. However, several approximate methods offer reasonable estimates:
1. Slater's Rules: This empirical method provides a relatively simple way to estimate Z<sub>eff</sub>. It assigns shielding constants to different groups of electrons based on their orbital type and location. The formula is:
Z<sub>eff</sub> = Z - S
where:
- Z = atomic number (number of protons)
- S = shielding constant (sum of contributions from other electrons)
Slater's rules provide specific weighting factors for electrons in different shells and subshells, accounting for the varying shielding effectiveness of s, p, d, and f electrons.
2. More sophisticated methods: For more accurate calculations, sophisticated quantum mechanical methods like Hartree-Fock calculations or Density Functional Theory (DFT) are employed. These methods solve the Schrödinger equation (or its approximations) to obtain a detailed description of the electron distribution and, consequently, a precise Z<sub>eff</sub> value.
Periodic Trends in Effective Nuclear Charge
Effective nuclear charge exhibits predictable trends across the periodic table:
-
Across a period (left to right): Z<sub>eff</sub> increases. As you move across a period, the number of protons increases, while the shielding effect remains relatively constant within the same shell. This results in a stronger attraction between the nucleus and the outer electrons, leading to a higher Z<sub>eff</sub>.
-
Down a group (top to bottom): Z<sub>eff</sub> increases only slightly. While both the number of protons and the number of shielding electrons increase down a group, the increase in shielding is proportionally greater than the increase in nuclear charge. Consequently, the increase in Z<sub>eff</sub> is less pronounced than the increase in Z. The addition of inner electron shells effectively shields the outer electrons from the increased nuclear charge.
What is Ionization Energy (IE)?
Ionization energy is the minimum energy required to remove an electron from a neutral gaseous atom in its ground state. This process forms a positively charged ion (cation). It’s a crucial property that reflects the strength of the atom's hold on its electrons. A higher ionization energy indicates a stronger attraction between the nucleus and the electron, making it more difficult to remove the electron.
Successive Ionization Energies
An atom can undergo successive ionization steps. The first ionization energy (IE<sub>1</sub>) corresponds to the removal of the first electron, the second ionization energy (IE<sub>2</sub>) to the removal of the second, and so on. Each successive ionization energy is generally higher than the previous one because removing an electron leaves a more positively charged ion, which holds onto the remaining electrons more tightly. The large jumps in ionization energies often indicate the removal of an electron from a different shell.
Measuring Ionization Energy
Ionization energy is typically measured experimentally using techniques such as photoelectron spectroscopy. In this technique, a beam of photons with known energy is directed at gaseous atoms. If the photon energy exceeds the ionization energy, an electron is ejected, and its kinetic energy can be measured. From this, the ionization energy can be calculated.
Periodic Trends in Ionization Energy
Ionization energy exhibits clear periodic trends, closely mirroring the trends in effective nuclear charge:
-
Across a period (left to right): Ionization energy generally increases. The increase in Z<sub>eff</sub> across a period leads to a stronger attraction between the nucleus and the outer electrons, resulting in a higher ionization energy. Exceptions may occur due to the relative stability of half-filled and fully filled subshells.
-
Down a group (top to bottom): Ionization energy generally decreases. The increase in atomic size and the increased shielding effect down a group weaken the attraction between the nucleus and the outer electrons, leading to a lower ionization energy.
The Interplay Between Effective Nuclear Charge and Ionization Energy
The relationship between effective nuclear charge and ionization energy is direct and fundamental. A higher Z<sub>eff</sub> implies a stronger attraction between the nucleus and the outermost electrons, making it more difficult to remove these electrons. Consequently, higher Z<sub>eff</sub> correlates with higher ionization energy.
The shielding effect plays a crucial role in this relationship. Increased shielding reduces Z<sub>eff</sub>, resulting in a weaker attraction and lower ionization energy. This is clearly seen in the periodic trends: the increase in shielding down a group counters the increase in nuclear charge, leading to a decrease in ionization energy despite the increasing number of protons.
Conversely, a decrease in shielding leads to a higher Z<sub>eff</sub> and, consequently, a higher ionization energy. This is evident in the increase in ionization energy across a period, where the shielding effect remains relatively constant while the nuclear charge increases.
Factors Influencing Ionization Energy Beyond Z<sub>eff</sub>
While Z<sub>eff</sub> is the primary determinant of ionization energy, other factors also contribute:
-
Electron-electron repulsions: The repulsions between electrons in the same shell or subshell can reduce the effective attraction of the nucleus and therefore slightly lower the ionization energy.
-
Penetration effect: Electrons in s orbitals penetrate closer to the nucleus than electrons in p, d, or f orbitals. This penetration effect leads to a higher Z<sub>eff</sub> for s electrons, resulting in a higher ionization energy for atoms where the outermost electrons are in s orbitals compared to those where the outermost electrons are in p orbitals within the same shell.
-
Electron configuration: The stability of half-filled and fully filled subshells affects ionization energy. Atoms with half-filled or fully filled subshells have slightly higher ionization energies than expected based solely on Z<sub>eff</sub> because these configurations are relatively stable.
-
Nuclear size and charge distribution: While the overall nuclear charge (Z) strongly influences the Z<sub>eff</sub>, the distribution of nuclear charge may also have a subtle influence on electron shielding and, subsequently, ionization energy.
Applications of Effective Nuclear Charge and Ionization Energy
The concepts of effective nuclear charge and ionization energy are not merely theoretical; they have numerous practical applications in various fields:
-
Predicting chemical reactivity: Ionization energy provides insights into the reactivity of elements. Elements with low ionization energies readily lose electrons and tend to be highly reactive metals. Elements with high ionization energies hold onto their electrons tightly and are less reactive.
-
Understanding bonding: The difference in ionization energies between atoms influences the nature of chemical bonds formed between them. For example, large differences in ionization energies promote the formation of ionic bonds, while smaller differences favor covalent bonds.
-
Spectroscopy: Ionization energy is directly linked to spectral lines observed in atomic spectroscopy. The energy required to ionize an atom can be determined by analyzing the wavelengths of light absorbed or emitted during electronic transitions.
-
Materials science: Understanding ionization energy is crucial in designing and synthesizing new materials with desired properties, like conductivity or reactivity.
Conclusion
Effective nuclear charge and ionization energy are two intertwined concepts that are essential for a complete understanding of atomic structure and chemical behavior. While Z<sub>eff</sub> is a crucial factor determining the magnitude of ionization energy, other factors also play a role. The periodic trends in both properties are well-established and highly valuable in predicting and interpreting chemical phenomena. The ability to calculate and understand these properties is critical in various branches of science and engineering, contributing to advancements in fields ranging from materials science to chemical reactivity. Further research continuously refines our understanding of these fundamental concepts and their subtle nuances.
Latest Posts
Latest Posts
-
How To Do Bohr Rutherford Diagrams
May 12, 2025
-
Is Milk Pure Substance Or Mixture
May 12, 2025
-
Power Series Of 1 1 X
May 12, 2025
-
Is Boron Trifluoride Polar Or Nonpolar
May 12, 2025
-
Which Point Of The Beam Experiences The Most Compression
May 12, 2025
Related Post
Thank you for visiting our website which covers about Effective Nuclear Charge And Ionization Energy . We hope the information provided has been useful to you. Feel free to contact us if you have any questions or need further assistance. See you next time and don't miss to bookmark.