How Do Cam Plants Minimize Photorespiration
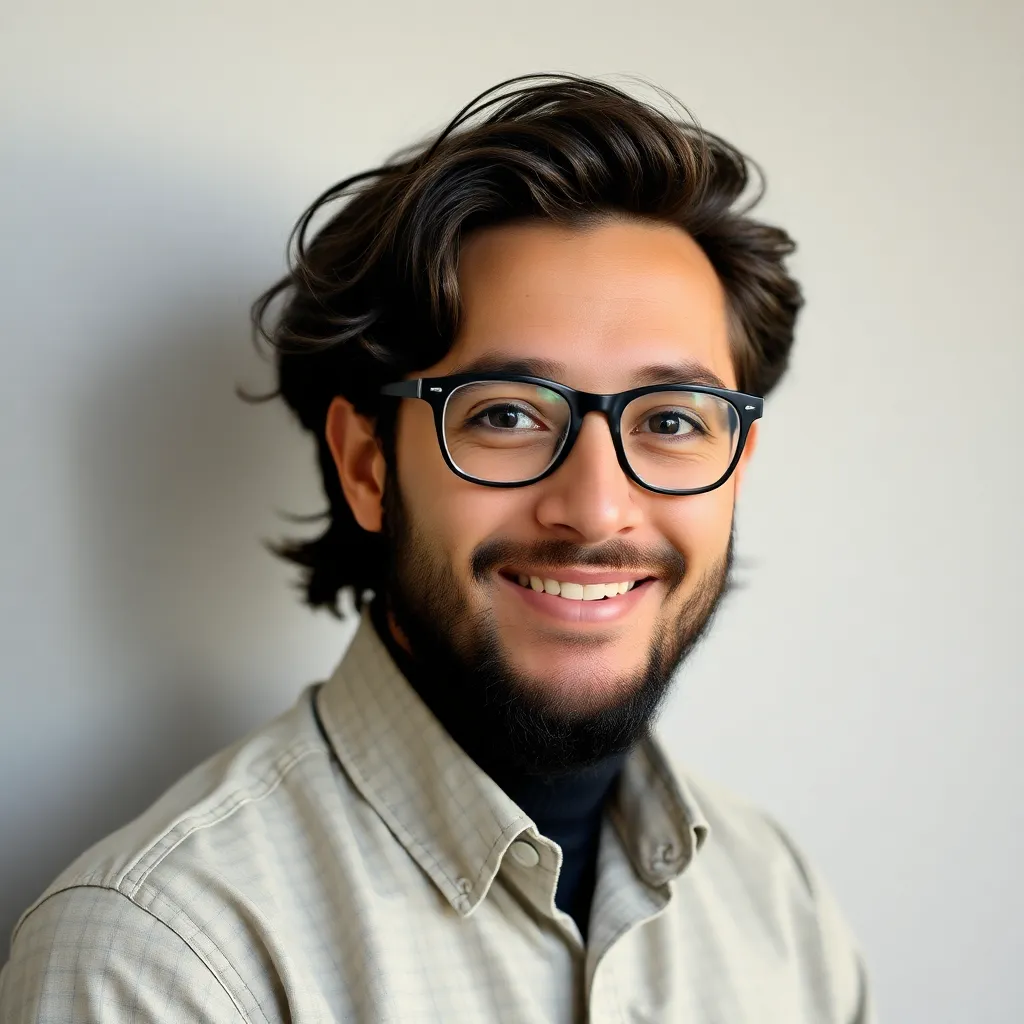
Muz Play
Apr 10, 2025 · 6 min read

Table of Contents
How Do CAM Plants Minimize Photorespiration?
Photorespiration is a metabolic process that occurs in plants, resulting in a decrease in photosynthetic efficiency. It's essentially a wasteful process where the enzyme RuBisCO, crucial for carbon fixation in photosynthesis, mistakenly binds with oxygen instead of carbon dioxide. This leads to the release of CO2 and a net loss of energy. While C3 and C4 plants have evolved different mechanisms to combat photorespiration, CAM (Crassulacean Acid Metabolism) plants take a unique approach, particularly effective in arid and semi-arid environments. This article delves deep into the strategies employed by CAM plants to minimize photorespiration and maximize their photosynthetic output in challenging conditions.
Understanding Photorespiration and its Consequences
Before exploring the CAM pathway's ingenious solution, it's crucial to understand the problem photorespiration poses. In the Calvin cycle, the primary process of carbon fixation in photosynthesis, RuBisCO catalyzes the carboxylation of ribulose-1,5-bisphosphate (RuBP), a five-carbon sugar. This reaction incorporates CO2 into the plant's metabolic pathways, leading to the production of carbohydrates. However, RuBisCO's active site can also bind with oxygen (O2), leading to photorespiration.
This oxygenation of RuBP produces phosphoglycolate, a two-carbon compound that is metabolically useless. Recovering the carbon from phosphoglycolate requires a series of reactions that consume ATP and NADPH, the energy currencies of the cell, ultimately leading to a net loss of energy and carbon. This is especially problematic in hot, dry conditions where stomata (tiny pores on leaves) close to conserve water, reducing CO2 uptake and increasing O2 concentration within the leaf. This heightened O2:CO2 ratio favors RuBisCO's oxygenase activity, exacerbating photorespiration.
The CAM Pathway: A Temporal Separation of Carbon Fixation and the Calvin Cycle
CAM plants, named after the Crassulaceae family where this metabolic pathway was first discovered, have evolved a remarkable solution to minimize photorespiration in water-stressed environments. Instead of spatially separating carbon fixation and the Calvin cycle as in C4 plants, CAM plants achieve this separation temporally, over the course of a day and night.
This temporal separation involves two distinct phases:
1. Night-time CO2 Uptake and Storage:
During the cool night, when transpiration rates are lower, CAM plants open their stomata. This allows them to take up atmospheric CO2. However, instead of directly entering the Calvin cycle, the CO2 is immediately converted into malic acid, a four-carbon organic acid, through a process involving phosphoenolpyruvate (PEP) carboxylase and other enzymes. This PEP carboxylase enzyme has a high affinity for CO2 and doesn't bind with oxygen, so the CO2 fixation is highly efficient. The malic acid is then stored in vacuoles, specialized compartments within the plant cells. This stage effectively concentrates CO2 within the plant overnight.
2. Day-time CO2 Release and Calvin Cycle:
During the day, when temperatures are high and light intensity is high, the stomata of CAM plants remain closed to reduce water loss. The malic acid stored in the vacuoles is decarboxylated, releasing CO2 into the cytoplasm. This internal CO2 concentration significantly increases compared to the external concentration, creating a CO2-rich environment within the cell. This high internal CO2 concentration then favors the carboxylase activity of RuBisCO, maximizing carbon fixation in the Calvin cycle while minimizing oxygenase activity and the wasteful process of photorespiration.
Key Enzymes and Processes in CAM Photosynthesis
The success of the CAM pathway depends on several key enzymes and processes:
-
PEP Carboxylase: This enzyme plays a pivotal role in the initial fixation of CO2 at night. Its high affinity for CO2 allows efficient carbon uptake, even at low CO2 concentrations.
-
Malic Enzyme: This enzyme is responsible for the decarboxylation of malic acid during the day, releasing CO2 for use in the Calvin cycle. Different types of malic enzymes exist, leading to variations in the CAM pathway.
-
Vacuolar Compartmentalization: The vacuole acts as a crucial storage compartment for malic acid during the night. Its large size and acidic pH enable efficient storage and release of malic acid.
-
Tight Regulation of Stomatal Opening and Closing: The precise control of stomatal opening and closing is essential to prevent excessive water loss while allowing sufficient CO2 uptake at night.
-
Metabolic Regulation: The entire process is tightly regulated by light, temperature, and water availability, ensuring that the pathway adapts to environmental changes.
Variations within the CAM Pathway
While the core principle of temporal separation is consistent, several variations exist within the CAM pathway, reflecting adaptations to diverse environmental conditions. These variations include:
-
Facultative CAM: Some plants can switch between C3 and CAM photosynthesis depending on environmental conditions. They may exhibit CAM characteristics under water stress but revert to C3 photosynthesis under favorable conditions.
-
CAM-cycling: This type of CAM involves a partial separation of carbon fixation and the Calvin cycle, with some overlap. It's found in plants with a less pronounced separation between day and night processes.
-
Variations in acid storage: The quantity and type of organic acids stored in the vacuoles can differ based on the specific plant species and the prevailing environmental conditions.
Advantages and Limitations of CAM Photosynthesis
The CAM pathway offers significant advantages, particularly in arid and semi-arid environments:
-
Reduced water loss: By opening stomata only at night, CAM plants significantly reduce water loss through transpiration.
-
Minimized photorespiration: The high internal CO2 concentration generated during the day favors the carboxylase activity of RuBisCO, thereby minimizing photorespiration.
-
Enhanced carbon fixation efficiency: The efficient capture and utilization of CO2 allow CAM plants to thrive in environments where water is scarce.
However, the CAM pathway also has limitations:
-
Slower growth rates: Compared to C3 and C4 plants, CAM plants generally exhibit slower growth rates due to the temporal separation of metabolic processes.
-
Limited CO2 uptake: The amount of CO2 that can be fixed overnight is limited by the storage capacity of the vacuoles.
-
Sensitivity to environmental fluctuations: Variations in temperature, light, and water availability can affect the efficiency of the CAM pathway.
Ecological Significance and Applications
CAM plants are found in a wide range of arid and semi-arid habitats, including deserts, rocky outcrops, and coastal regions. They play a significant role in the functioning of these ecosystems, contributing to primary productivity and supporting diverse animal communities. The unique physiology of CAM plants also holds potential for applications in agriculture and biotechnology. For example, researchers are exploring ways to introduce CAM characteristics into C3 crops to improve water-use efficiency and drought tolerance. Furthermore, the understanding of the CAM pathway can inform the development of more efficient and sustainable agricultural practices in water-scarce regions.
Conclusion
CAM plants have evolved a sophisticated and efficient strategy to minimize photorespiration and maximize photosynthetic productivity in water-limited environments. The temporal separation of carbon fixation and the Calvin cycle, coupled with efficient CO2 storage and release, allows these plants to thrive under conditions where other plants struggle. The continued study of CAM photosynthesis holds immense potential for improving crop productivity and adapting agriculture to the challenges of climate change. Understanding the intricate details of this fascinating metabolic pathway is crucial not only for expanding our knowledge of plant biology but also for addressing critical challenges in food security and environmental sustainability.
Latest Posts
Latest Posts
-
A Molecule With Partially Charged Areas
Apr 18, 2025
-
Label The Structures Of The Internal View Of The Skull
Apr 18, 2025
-
An Angle Measure 36 Degrees So Its Classified As
Apr 18, 2025
-
What Does True Breeding Mean In Biology
Apr 18, 2025
-
Which Way Do Electrons Flow In A Galvanic Cell
Apr 18, 2025
Related Post
Thank you for visiting our website which covers about How Do Cam Plants Minimize Photorespiration . We hope the information provided has been useful to you. Feel free to contact us if you have any questions or need further assistance. See you next time and don't miss to bookmark.