Matter Is Not Created Or Destroyed But It Can
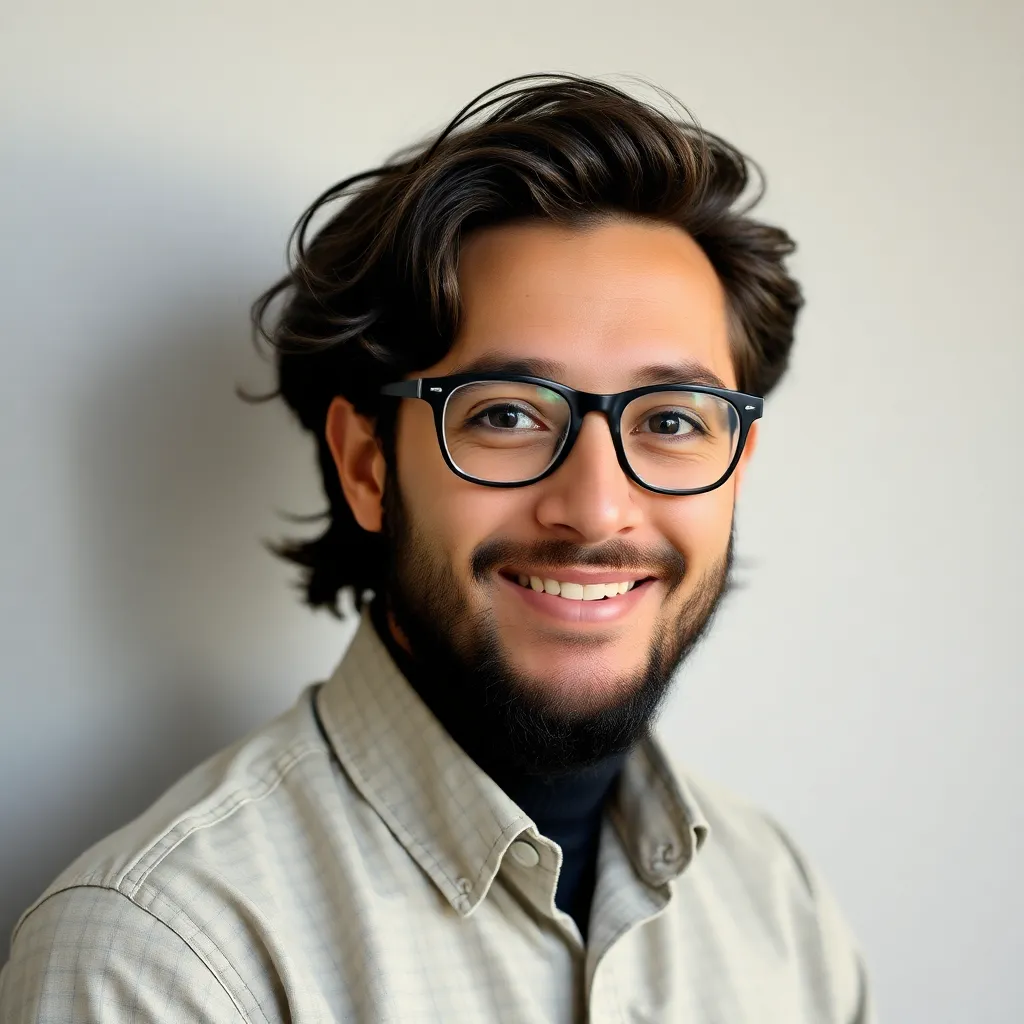
Muz Play
May 11, 2025 · 6 min read
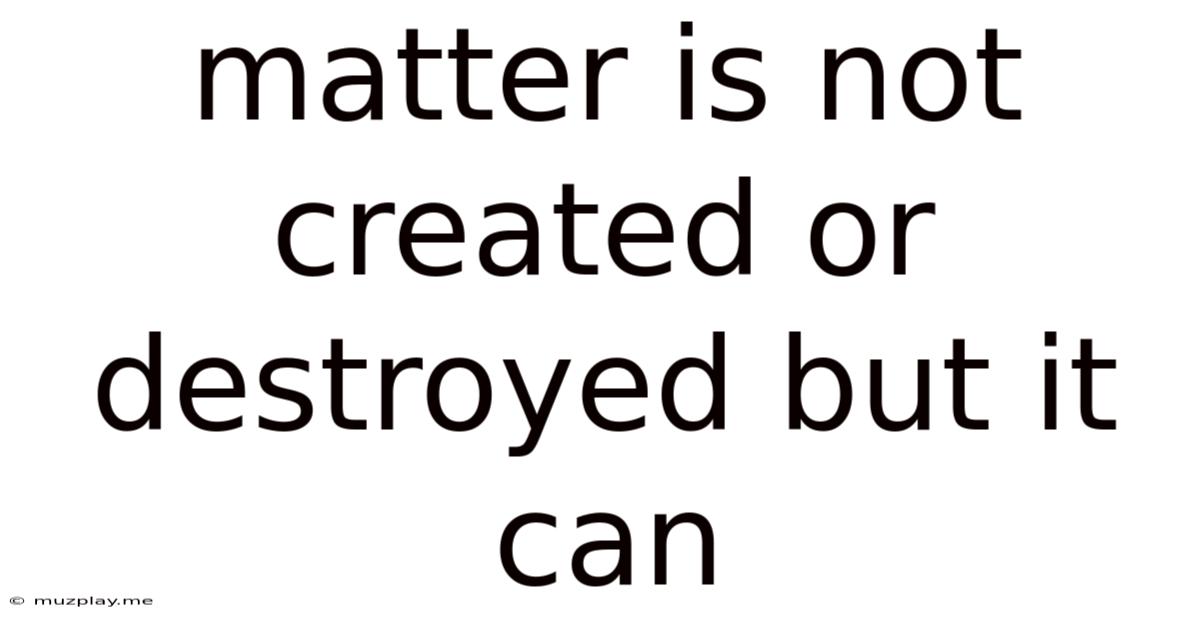
Table of Contents
Matter is Not Created or Destroyed, But It Can… Transform!
The enduring principle of conservation of mass, a cornerstone of classical physics, famously states that matter cannot be created or destroyed. While seemingly straightforward, this statement requires nuance and a deeper understanding of the universe's fundamental laws. It's not that matter vanishes into thin air, but rather that it undergoes transformations, shifting its form and configuration in intricate and fascinating ways. This article delves into the complexities of this principle, exploring the various ways matter changes state, phase, and even fundamental composition while upholding the principle of conservation.
The Classical Understanding: Conservation of Mass
Before we explore the exceptions and nuances, let's solidify the foundation. The principle of conservation of mass, in its simplest form, asserts that the total mass in a closed system remains constant over time. This means that in any physical or chemical process, the mass of the reactants (the starting materials) will always equal the mass of the products (the resulting substances). This principle was crucial in the development of early chemistry, allowing scientists to balance chemical equations and predict the outcomes of reactions.
Think of a simple chemical reaction like burning wood. The wood, along with the oxygen in the air, undergoes combustion. The resulting products – ash, smoke, and gases – might appear to have less mass than the original wood and oxygen. However, if we meticulously collected all the products, including the invisible gases, we would find that the total mass remains the same. The mass hasn't been destroyed; it's simply been rearranged into different forms.
Limitations of the Classical View
However, the classical understanding of conservation of mass faces limitations when considering the complexities of modern physics. The limitations become apparent when we consider the interplay between mass and energy, as outlined by Einstein's famous equation, E=mc².
Einstein's E=mc²: Mass-Energy Equivalence
Einstein's groundbreaking equation revolutionized our understanding of mass and energy, demonstrating their fundamental interconnectedness. The equation reveals that mass and energy are essentially interchangeable; they are two sides of the same coin. A small amount of mass can be converted into a tremendous amount of energy, and vice versa. This has profound implications for the conservation of mass.
Nuclear Reactions: Mass Conversion to Energy
Nuclear reactions, such as nuclear fission (splitting of atoms) and nuclear fusion (combining of atoms), directly illustrate the mass-energy equivalence. In these reactions, a small amount of mass is converted into a substantial amount of energy. For example, in nuclear fission of uranium, a small fraction of the uranium's mass is converted into energy, resulting in a massive release of energy – the principle behind nuclear power plants and atomic bombs. The total mass-energy of the system remains constant, but the mass of the individual components changes.
Particle Physics: Mass and Antimatter Annihilation
The world of particle physics further complicates the notion of strict mass conservation. The discovery of antimatter, particles with the same mass but opposite charge as their matter counterparts, introduces another layer of complexity. When matter and antimatter collide, they annihilate each other, converting their entire mass into energy in the form of photons (light). This process dramatically highlights the interconversion of mass and energy.
Phase Transitions and State Changes
Even without nuclear reactions or particle annihilation, matter undergoes constant transformations without violating the principle of mass conservation. Phase transitions, such as the changes between solid, liquid, and gas states, are prime examples.
Solid, Liquid, Gas: A Matter of Arrangement
The difference between ice (solid), water (liquid), and steam (gas) is not in the number of water molecules but in their arrangement and the energy they possess. Heating ice to melt it or boil water into steam requires energy input. This energy doesn't create additional water molecules; it simply increases the kinetic energy of the existing molecules, changing their arrangement and thus their phase. The total mass remains the same.
Sublimation and Deposition: Direct Transitions
Sublimation and deposition represent further examples of phase transitions without altering the total mass. Sublimation is the direct transition from solid to gas (dry ice turning into carbon dioxide gas), while deposition is the reverse process (frost forming on a cold surface). Again, no matter is created or destroyed; it merely changes its phase.
Chemical Reactions: Rearrangement of Atoms
Chemical reactions, unlike nuclear reactions, do not involve changes in the nuclei of atoms. They involve the rearrangement of atoms to form new molecules. In these reactions, the total number of atoms of each element remains constant. The mass of the reactants will always equal the mass of the products, provided that all products are accounted for.
Combustion and Synthesis: Examples of Chemical Changes
Combustion, as discussed earlier, is an example of a chemical reaction. Other examples include synthesis reactions (combining simpler substances to form more complex ones) and decomposition reactions (breaking down complex substances into simpler ones). In all these cases, while the molecular structure changes, the total mass remains conserved.
The Modern Understanding: Conservation of Mass-Energy
The modern understanding transcends the limitations of the classical view. We now understand that the total mass-energy of a closed system remains constant. While mass can be converted to energy and vice versa, the total quantity of mass-energy remains unchanged. This more accurate statement encompasses both classical and relativistic physics.
Expanding the Scope: Cosmology and the Big Bang
Even at the cosmological level, the principle of mass-energy conservation holds true, although its application necessitates a complex understanding of dark matter and dark energy. The Big Bang theory, the prevailing cosmological model for the universe's origin, proposes that the universe began from an incredibly dense and hot singularity. While the initial conditions remain a subject of ongoing research, the total mass-energy of the universe is believed to have remained constant since the Big Bang.
Conclusion: Transformation, Not Destruction
In conclusion, the statement "matter is not created or destroyed" requires a refined perspective. Matter itself is not created or destroyed; instead, it undergoes transformations, changing its state, phase, and even its fundamental composition. Einstein's E=mc² elegantly highlights the interplay between mass and energy, demonstrating that mass can be converted into energy and vice versa. The total mass-energy of a closed system, however, remains constant, upholding the fundamental principle of conservation. Understanding this principle is critical across multiple scientific disciplines, from chemistry and physics to cosmology and beyond. The universe is a dynamic system of constant transformation, but the underlying principle of conservation of mass-energy provides a vital framework for understanding its workings.
Latest Posts
Latest Posts
-
How Does A Positive Ion Form
May 12, 2025
-
A Pictorial Representation Of An Electronic Configuration Is Shown
May 12, 2025
-
What Does Area Under The Curve Mean In Statistics
May 12, 2025
-
Current Voltage And Resistance Worksheet Answers Unit 9 3
May 12, 2025
-
What Is The Difference Between A Open And Closed System
May 12, 2025
Related Post
Thank you for visiting our website which covers about Matter Is Not Created Or Destroyed But It Can . We hope the information provided has been useful to you. Feel free to contact us if you have any questions or need further assistance. See you next time and don't miss to bookmark.