Microscopic Anatomy Of A Muscle Fiber
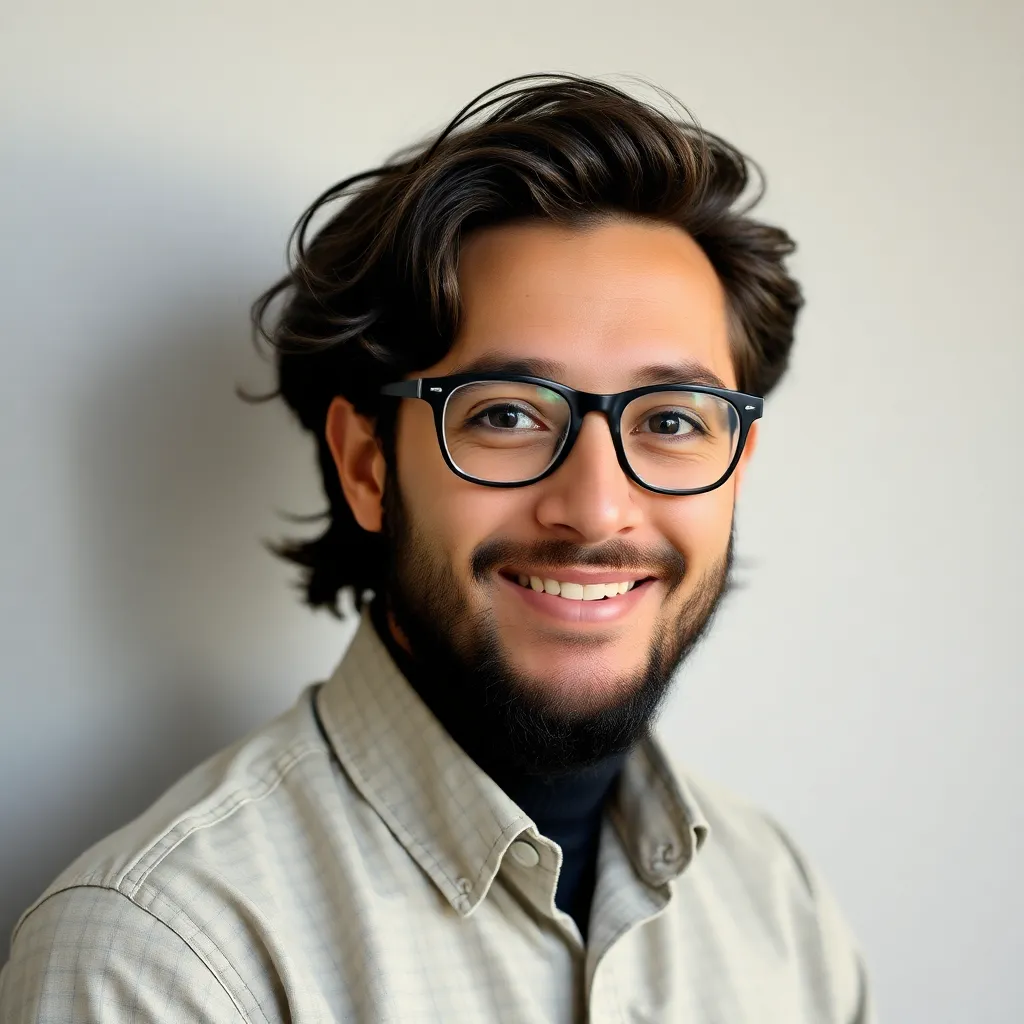
Muz Play
Apr 01, 2025 · 7 min read
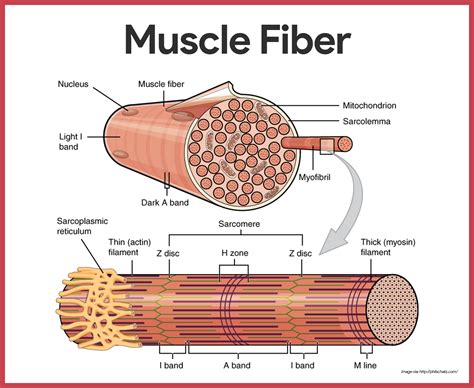
Table of Contents
Microscopic Anatomy of a Muscle Fiber: A Deep Dive
The human body is a marvel of engineering, and nowhere is this more evident than in the intricate workings of our muscles. These dynamic tissues, responsible for movement, posture, and even internal organ function, are composed of millions of individual muscle fibers. Understanding the microscopic anatomy of these fibers is crucial to grasping how muscles contract, generate force, and adapt to training and injury. This article delves into the detailed structure of a muscle fiber, exploring its components and their roles in muscle function.
The Structure of a Muscle Fiber: A Hierarchical Organization
A muscle fiber, also known as a muscle cell, is a highly specialized elongated cylindrical cell. It's not just a simple cell, though; it's a remarkably complex structure organized in a hierarchical manner, exhibiting a fascinating interplay of proteins and organelles. This organization allows for efficient force generation and transmission. The key components include:
1. The Sarcolemma: The Muscle Fiber's Membrane
The sarcolemma is the plasma membrane that encloses the muscle fiber. More than just a barrier, it plays a crucial role in muscle excitation and contraction. Its specialized features include:
- Transverse Tubules (T-tubules): These invaginations of the sarcolemma extend deep into the muscle fiber, carrying action potentials from the surface to the interior. This rapid transmission ensures that the entire fiber contracts simultaneously.
- Motor End Plate: The specialized region of the sarcolemma where a motor neuron synapses with the muscle fiber. This is the site of neuromuscular transmission, where the nerve impulse triggers muscle contraction.
2. The Sarcoplasm: The Muscle Fiber's Cytoplasm
The sarcoplasm is the cytoplasm of the muscle fiber. It contains a unique blend of organelles and molecules crucial for muscle function:
- Myofibrils: These are the cylindrical structures that run the length of the muscle fiber and are the primary contractile units. They're composed of repeating units called sarcomeres, which we'll examine in detail below.
- Sarcoplasmic Reticulum (SR): This specialized endoplasmic reticulum is responsible for regulating calcium ion (Ca²⁺) concentration within the muscle fiber. Ca²⁺ is absolutely essential for muscle contraction, and the SR acts as a calcium reservoir, releasing it upon stimulation and reabsorbing it to terminate contraction.
- Mitochondria: These powerhouses of the cell provide the ATP (adenosine triphosphate) necessary for muscle contraction. Muscle fibers are highly metabolically active, requiring a substantial energy supply. The abundance of mitochondria reflects this high energy demand.
- Glycogen Granules: These store glycogen, a readily available source of glucose for energy production. Glycogenolysis, the breakdown of glycogen, provides a quick energy boost during muscle activity.
- Myoglobin: This oxygen-binding protein stores oxygen within the muscle fiber, providing a ready reserve for aerobic respiration. Myoglobin gives muscle tissue its characteristic red color.
3. Myofibrils: The Contractile Machinery
Myofibrils are the fundamental contractile units of the muscle fiber. Their highly organized structure is responsible for the striated appearance of skeletal muscle under a microscope. The key structural components of a myofibril include:
- Sarcomeres: These are the repeating units of the myofibril, ranging from 2 to 3 µm in length. Each sarcomere is delimited by Z-lines (or Z-discs), dense protein structures. The sarcomere is the functional unit of muscle contraction.
- Thick Filaments (Myosin): These are located in the center of the sarcomere, forming the A band (anisotropic band). Each thick filament consists of hundreds of myosin molecules, each with a head and tail. The myosin heads bind to actin filaments, driving muscle contraction.
- Thin Filaments (Actin): These extend from the Z-lines toward the center of the sarcomere, overlapping with the thick filaments. Actin filaments are composed primarily of actin, tropomyosin, and troponin, proteins that regulate the interaction between actin and myosin.
- M-line: This is a structural protein in the center of the sarcomere, anchoring the thick filaments.
- H-zone: The region in the center of the sarcomere that contains only thick filaments, appearing lighter under the microscope. This zone narrows during muscle contraction.
- I-band (isotropic band): The lighter band at either end of the sarcomere containing only thin filaments. This band shortens during muscle contraction.
The Molecular Mechanisms of Muscle Contraction: The Sliding Filament Theory
The sliding filament theory elegantly explains how muscle contraction occurs at the molecular level. It postulates that muscle contraction results from the sliding of thin filaments over thick filaments within the sarcomere. This process is driven by the interaction between actin and myosin:
-
Nerve Impulse and Calcium Release: A nerve impulse triggers the release of acetylcholine at the neuromuscular junction, initiating an action potential that spreads along the sarcolemma and into the T-tubules. This triggers the release of Ca²⁺ from the SR into the sarcoplasm.
-
Calcium Binding and Cross-Bridge Formation: The released Ca²⁺ binds to troponin, causing a conformational change in tropomyosin. This exposes the myosin-binding sites on actin. Myosin heads then bind to these sites, forming cross-bridges.
-
Power Stroke: The myosin heads undergo a conformational change, pivoting and pulling the thin filaments towards the center of the sarcomere. This is the power stroke, generating force.
-
ATP Binding and Detachment: ATP binds to the myosin head, causing it to detach from actin. Hydrolysis of ATP provides the energy for the myosin head to return to its original conformation.
-
Cross-Bridge Cycling: This cycle of cross-bridge formation, power stroke, ATP binding, and detachment repeats multiple times, resulting in the sliding of thin filaments over thick filaments and the shortening of the sarcomere.
-
Calcium Reabsorption: When the nerve impulse ceases, Ca²⁺ is actively pumped back into the SR, causing tropomyosin to cover the myosin-binding sites on actin. Muscle contraction ceases.
Types of Muscle Fibers: Variations in Structure and Function
Not all muscle fibers are created equal. Skeletal muscle fibers are classified into different types based on their contractile and metabolic properties:
1. Type I (Slow-Twitch) Fibers: Endurance Champions
These fibers are characterized by their slow contraction speed, high oxidative capacity, and resistance to fatigue. They rely primarily on aerobic respiration for energy production and are rich in mitochondria and myoglobin. Type I fibers are well-suited for endurance activities.
2. Type IIa (Fast-Twitch Oxidative) Fibers: Versatile Performers
These fibers contract faster than Type I fibers and have a moderate oxidative capacity. They can utilize both aerobic and anaerobic metabolism for energy production and exhibit intermediate fatigue resistance. Type IIa fibers are well-suited for activities requiring both speed and endurance.
3. Type IIx (Fast-Twitch Glycolytic) Fibers: Powerhouses
These fibers contract rapidly and have a high glycolytic capacity, relying primarily on anaerobic metabolism for energy production. They fatigue quickly but generate significant power. Type IIx fibers are ideal for short bursts of intense activity.
4. Type IIb (Fast-Twitch Glycolytic) Fibers: The Fastest
These are the fastest contracting muscle fibers. They rely almost exclusively on anaerobic metabolism for energy production and are highly prone to fatigue. While less common in humans than other fiber types, they are important for extremely powerful, short-duration activities.
The Importance of Understanding Muscle Fiber Anatomy
Understanding the microscopic anatomy of muscle fibers is essential for various reasons:
-
Exercise Physiology: Knowledge of muscle fiber types and their metabolic properties informs exercise prescription and training programs. Targeted training can lead to adaptations in muscle fiber composition, improving athletic performance.
-
Muscle Diseases: Many muscle diseases are associated with structural or functional abnormalities in muscle fibers. Microscopic examination of muscle biopsies is crucial for diagnosing and managing these conditions.
-
Regenerative Medicine: Research on muscle regeneration focuses on understanding the mechanisms of muscle fiber repair and growth. This knowledge may lead to innovative therapies for muscle injuries and diseases.
-
Bioengineering: Understanding muscle fiber mechanics is crucial for developing artificial muscles and other bio-inspired technologies.
Conclusion: A Complex System for Efficient Movement
The microscopic anatomy of a muscle fiber is a testament to the intricate design of the human body. The highly organized structure of myofibrils, the sophisticated interplay of proteins, and the diverse types of muscle fibers all contribute to the remarkable ability of muscles to generate force, power movement, and adapt to various demands. Further research into the complexities of muscle fiber structure and function promises to unlock even greater understanding of human physiology and pave the way for advances in medicine, sports science, and bioengineering.
Latest Posts
Latest Posts
-
What Is The Conflict Of The Play
Apr 02, 2025
-
The Cell Is The Basic Unit Of
Apr 02, 2025
-
Comparison Of Somatic And Autonomic Nervous Systems Concept Map
Apr 02, 2025
-
How To Convert Molecules To Atoms
Apr 02, 2025
-
Ejemplo De Diagrama De Cuerpo Libre
Apr 02, 2025
Related Post
Thank you for visiting our website which covers about Microscopic Anatomy Of A Muscle Fiber . We hope the information provided has been useful to you. Feel free to contact us if you have any questions or need further assistance. See you next time and don't miss to bookmark.