Tertiary Protein Structure Results Mainly From Which Interaction Or Bonding
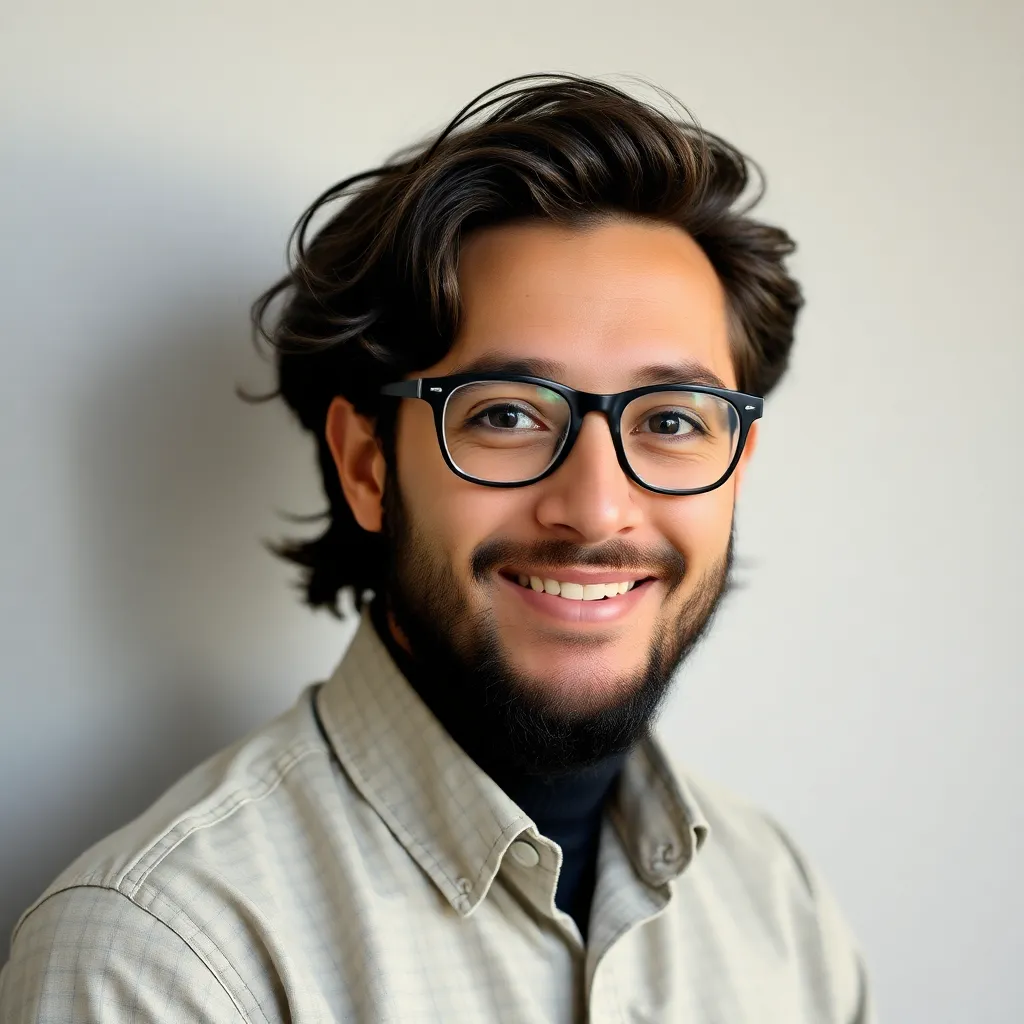
Muz Play
Apr 20, 2025 · 6 min read

Table of Contents
Tertiary Protein Structure: A Deep Dive into the Interactions that Shape Functionality
Tertiary protein structure represents the three-dimensional arrangement of a polypeptide chain, a crucial determinant of its biological function. Unlike primary (amino acid sequence) and secondary (local folding patterns like alpha-helices and beta-sheets) structures, the tertiary structure encompasses the overall spatial organization of the entire protein, including the positioning of its secondary structure elements relative to each other. This intricate folding is not random; it's driven by a complex interplay of various non-covalent interactions and, in some cases, covalent bonds. Understanding these interactions is paramount to comprehending protein function and misfolding-related diseases. This article will delve deeply into the primary forces shaping tertiary protein structure.
The Dominant Forces: Non-Covalent Interactions
The tertiary structure of a protein is primarily stabilized by a suite of weak, non-covalent interactions. While individually weak, the cumulative effect of numerous such interactions across the protein's vast surface area leads to a remarkably stable and specific three-dimensional conformation. These forces include:
1. Hydrophobic Interactions: The Driving Force
Arguably the most significant driving force in protein folding is the hydrophobic effect. Amino acid side chains can be broadly classified as hydrophobic (nonpolar) or hydrophilic (polar). During folding, the protein attempts to minimize its unfavorable contact with water. This results in the clustering of hydrophobic residues in the protein's interior, away from the aqueous environment. This "hydrophobic core" is stabilized by van der Waals forces between tightly packed nonpolar side chains.
-
Mechanism: Water molecules surrounding hydrophobic residues form highly ordered structures, a thermodynamically unfavorable state. By burying the hydrophobic residues within the protein, the system increases its entropy (disorder) by releasing these ordered water molecules. This entropic gain is a major contributor to the folding process.
-
Significance: The hydrophobic effect is a powerful force that dictates the overall shape and compactness of the protein, pushing the hydrophobic amino acids to the core and exposing the hydrophilic residues to the surrounding solvent. It is crucial for defining the core structure and stability.
2. Hydrogen Bonds: Stabilizing Secondary and Tertiary Structures
Hydrogen bonds play a crucial role in both secondary and tertiary structures. In the tertiary structure, they contribute to the overall stabilization of the folded conformation by linking polar side chains or backbone atoms. Hydrogen bonds occur between an electronegative atom (like oxygen or nitrogen) and a hydrogen atom covalently bonded to another electronegative atom.
-
Specificity: The strength and directionality of hydrogen bonds contribute to the precise arrangement of secondary structural elements and the interactions between distant parts of the polypeptide chain.
-
Importance: While individually weaker than covalent bonds, the numerous hydrogen bonds formed across the protein contribute significantly to its overall stability and precise folding. They are particularly important in stabilizing alpha-helices and beta-sheets, which further define the tertiary structure.
3. Ionic Interactions (Salt Bridges): Electrostatic Attraction
Ionic interactions, or salt bridges, arise between oppositely charged amino acid side chains. For example, a positively charged lysine residue can form an ionic bond with a negatively charged aspartate residue. These interactions are relatively strong and contribute significantly to the protein's stability.
-
Environment Dependence: The strength of ionic interactions is highly sensitive to the surrounding environment, particularly the dielectric constant of the solvent. In the protein's hydrophobic core, ionic interactions are generally stronger because the low dielectric constant reduces the shielding effect of water.
-
Specificity: The specific location and arrangement of ionic interactions greatly contribute to the unique shape and properties of the protein. Disruption of these interactions can lead to significant changes in protein structure and function.
4. Van der Waals Forces: Weak but Numerous
Van der Waals forces are weak, short-range interactions that arise from temporary fluctuations in electron distribution around atoms. While individually weak, the cumulative effect of numerous van der Waals interactions between closely packed atoms in the protein's core can contribute significantly to its overall stability.
-
Optimizing Packing: These forces contribute to the close packing of atoms within the protein's interior, optimizing the hydrophobic core's density and stability.
-
Non-specificity: Unlike hydrogen bonds or ionic interactions, van der Waals forces are relatively non-specific and depend primarily on the proximity of atoms. Their contribution to protein stability stems from their sheer number.
The Minor Player: Covalent Bonds
While non-covalent interactions dominate in shaping tertiary structure, covalent bonds play a supporting, but important, role. The most prominent example is:
1. Disulfide Bonds: Stabilizing Cross-Links
Disulfide bonds are covalent linkages formed between the thiol groups (-SH) of cysteine residues. These bonds are strong and significantly contribute to the protein's stability, particularly in proteins exposed to extracellular environments.
-
Oxidative Formation: Disulfide bonds are typically formed through an oxidative process, often occurring in the endoplasmic reticulum of eukaryotic cells.
-
Structural Constraints: They create cross-links within the polypeptide chain, imposing structural constraints and stabilizing the three-dimensional arrangement. The presence and positioning of disulfide bonds are crucial in defining the protein's final conformation and resistance to denaturation.
Factors Influencing Tertiary Structure
Several factors beyond the inherent interactions between amino acid residues influence the final tertiary structure of a protein:
-
Amino Acid Sequence: The primary structure, the linear sequence of amino acids, is the blueprint for the tertiary structure. The properties of individual amino acids (hydrophobic, hydrophilic, charged, etc.) dictate the interactions that will drive the folding process.
-
Chaperones: Molecular chaperones are proteins that assist in the correct folding of other proteins. They prevent aggregation of partially folded proteins and facilitate the formation of the native tertiary structure.
-
Environmental Factors: The surrounding environment, including temperature, pH, and the presence of ions, can significantly impact protein folding. Changes in these factors can lead to denaturation, or unfolding, of the protein.
-
Post-translational Modifications: Chemical modifications to amino acid residues after protein synthesis can also influence tertiary structure. For example, glycosylation (addition of sugar molecules) can affect protein folding and stability.
Consequences of Incorrect Tertiary Structure
The correct tertiary structure of a protein is crucial for its function. Misfolding can lead to loss of function or the acquisition of harmful properties. Many diseases, including Alzheimer's disease, Parkinson's disease, and cystic fibrosis, are associated with protein misfolding and aggregation.
Conclusion
The tertiary structure of a protein is a marvel of molecular engineering, a precisely folded conformation stabilized by a delicate balance of non-covalent interactions, with covalent bonds playing a supporting role. Understanding the intricacies of these interactions is fundamental to comprehending protein function and the pathogenesis of protein misfolding diseases. The hydrophobic effect serves as the primary driving force, while hydrogen bonds, ionic interactions, and van der Waals forces contribute to the stabilization of the folded state. Disulfide bonds add a layer of covalent stabilization, particularly in extracellular proteins. The intricate interplay of these forces, influenced by the amino acid sequence and environmental factors, leads to the unique three-dimensional arrangement that defines a protein's biological role. Further research continues to unravel the complexities of protein folding, with the ultimate goal of harnessing this knowledge for therapeutic applications and biotechnological advancements.
Latest Posts
Latest Posts
-
How To Find Frequency From Class Boundaries
Apr 20, 2025
-
Elements That Are Gases At Room Temp
Apr 20, 2025
-
Dna Makes A Good Molecule For Storing Information Because
Apr 20, 2025
-
Using Three Criteria For Double Displacement Reactions
Apr 20, 2025
-
The Three Major Cost Of Manufacturing A Product Are
Apr 20, 2025
Related Post
Thank you for visiting our website which covers about Tertiary Protein Structure Results Mainly From Which Interaction Or Bonding . We hope the information provided has been useful to you. Feel free to contact us if you have any questions or need further assistance. See you next time and don't miss to bookmark.