The Monomers Of Proteins Are Called
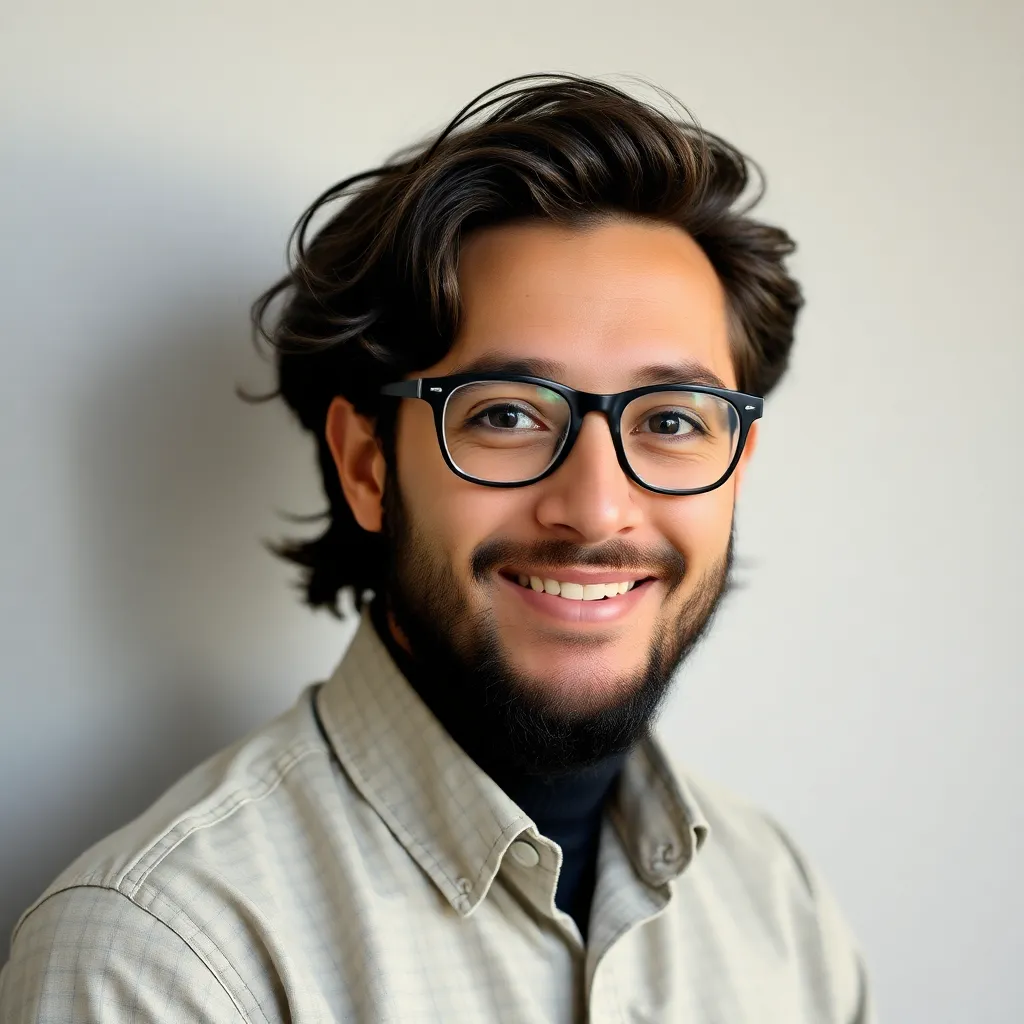
Muz Play
Mar 29, 2025 · 7 min read
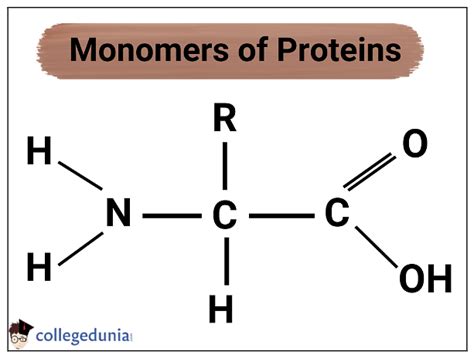
Table of Contents
The Monomers of Proteins Are Called: Amino Acids – A Deep Dive into Protein Structure and Function
Proteins are the workhorses of life, performing a vast array of functions crucial for cellular processes and overall organismal health. From catalyzing biochemical reactions as enzymes to providing structural support as components of the cytoskeleton, proteins are essential for virtually every aspect of biology. But what are these amazing molecules fundamentally made of? The answer lies in their basic building blocks: amino acids.
Understanding Amino Acids: The Building Blocks of Proteins
Amino acids are the monomers of proteins, meaning they are the individual units that link together to form the larger polymer, the protein. These monomers aren't simply strung together randomly; their specific sequence dictates the protein's unique three-dimensional structure and, consequently, its function.
The Basic Structure of an Amino Acid
Each amino acid shares a common core structure, consisting of:
-
A central carbon atom (α-carbon): This carbon atom is chiral, meaning it's bonded to four different groups. This chirality is crucial for the protein's overall structure and function.
-
An amino group (-NH₂): This group is basic and carries a positive charge at physiological pH.
-
A carboxyl group (-COOH): This group is acidic and carries a negative charge at physiological pH.
-
A hydrogen atom (-H): This is a simple hydrogen atom bonded to the α-carbon.
-
A variable side chain (R-group): This is the unique part of each amino acid, giving each its distinct chemical properties. The R-group can be anything from a simple hydrogen atom (as in glycine) to a complex aromatic ring structure (as in tryptophan). It is the diversity of these R-groups that accounts for the incredible variety of proteins found in nature.
The 20 Standard Amino Acids
There are 20 standard amino acids commonly found in proteins. These amino acids are encoded by the genetic code and are used by ribosomes to synthesize proteins. These 20 amino acids can be categorized based on the properties of their side chains:
1. Nonpolar, aliphatic amino acids: These amino acids have hydrophobic (water-repelling) side chains. Examples include:
- Glycine (Gly, G): The simplest amino acid, with a hydrogen atom as its R-group.
- Alanine (Ala, A): A methyl group (-CH₃) as its R-group.
- Valine (Val, V): A branched-chain aliphatic group.
- Leucine (Leu, L): A branched-chain aliphatic group.
- Isoleucine (Ile, I): A branched-chain aliphatic group, an isomer of leucine.
- Methionine (Met, M): Contains a thioether group (-CH₂SCH₃).
2. Aromatic amino acids: These amino acids have aromatic rings in their side chains, contributing to their hydrophobicity and ability to absorb UV light. Examples include:
- Phenylalanine (Phe, F): Contains a benzene ring.
- Tyrosine (Tyr, Y): Contains a benzene ring with a hydroxyl group (-OH).
- Tryptophan (Trp, W): Contains an indole ring.
3. Polar, uncharged amino acids: These amino acids have polar, but uncharged, side chains that can form hydrogen bonds. Examples include:
- Serine (Ser, S): Contains a hydroxyl group (-OH).
- Threonine (Thr, T): Contains a hydroxyl group (-OH).
- Cysteine (Cys, C): Contains a thiol group (-SH), which can form disulfide bonds.
- Asparagine (Asn, N): Contains an amide group (-CONH₂).
- Glutamine (Gln, Q): Contains an amide group (-CONH₂).
4. Positively charged (basic) amino acids: These amino acids have side chains that carry a positive charge at physiological pH. Examples include:
- Lysine (Lys, K): Contains an amino group (-NH₃⁺) at the end of its side chain.
- Arginine (Arg, R): Contains a guanidinium group.
- Histidine (His, H): Contains an imidazole ring.
5. Negatively charged (acidic) amino acids: These amino acids have side chains that carry a negative charge at physiological pH. Examples include:
- Aspartic acid (Asp, D): Contains a carboxyl group (-COO⁻).
- Glutamic acid (Glu, E): Contains a carboxyl group (-COO⁻).
From Amino Acids to Proteins: The Process of Protein Synthesis
The formation of a protein from its amino acid monomers is a complex process known as protein synthesis, which involves two main stages:
1. Transcription: DNA to mRNA
The genetic information for protein synthesis is stored in DNA. Transcription is the process of copying this information into a messenger RNA (mRNA) molecule. The mRNA molecule then carries the genetic code from the nucleus (in eukaryotes) to the ribosomes in the cytoplasm, where protein synthesis takes place.
2. Translation: mRNA to Protein
Translation is the process by which the genetic code in mRNA is used to synthesize a protein. Ribosomes read the mRNA sequence in codons (three-nucleotide units), each codon specifying a particular amino acid. Transfer RNA (tRNA) molecules bring the appropriate amino acids to the ribosome, where they are linked together by peptide bonds to form a polypeptide chain. This polypeptide chain then folds into a specific three-dimensional structure to become a functional protein.
The Importance of Amino Acid Sequence and Protein Structure
The sequence of amino acids in a protein, also known as the primary structure, dictates its higher-order structures and, ultimately, its function. The interactions between the amino acid side chains determine how the polypeptide chain folds. There are four levels of protein structure:
1. Primary Structure: The Amino Acid Sequence
The primary structure is simply the linear sequence of amino acids in the polypeptide chain. This sequence is determined by the genetic code and is crucial for determining the protein's subsequent folding.
2. Secondary Structure: Local Folding Patterns
The secondary structure refers to local folding patterns within the polypeptide chain, stabilized by hydrogen bonds between the backbone atoms. Common secondary structures include:
- α-helices: A right-handed coiled structure.
- β-sheets: Extended, pleated sheets formed by hydrogen bonds between adjacent polypeptide strands.
- Loops and turns: Irregular regions connecting α-helices and β-sheets.
3. Tertiary Structure: The Overall 3D Structure
The tertiary structure is the overall three-dimensional arrangement of the polypeptide chain, including the spatial relationships between all the amino acid residues. This structure is stabilized by a variety of interactions, including:
- Disulfide bonds: Covalent bonds between cysteine residues.
- Hydrogen bonds: Between polar side chains.
- Ionic bonds (salt bridges): Between charged side chains.
- Hydrophobic interactions: Between nonpolar side chains.
4. Quaternary Structure: Multiple Polypeptide Chains
Some proteins consist of multiple polypeptide chains, each with its own tertiary structure. The arrangement of these multiple chains is called the quaternary structure. Examples include hemoglobin, which consists of four polypeptide chains.
The Roles of Proteins in Biological Systems
The diverse functions of proteins stem from their varied structures and chemical properties. Some key roles of proteins include:
- Enzymes: Catalyze biochemical reactions, speeding up metabolic processes.
- Structural proteins: Provide support and shape to cells and tissues (e.g., collagen, keratin).
- Transport proteins: Carry molecules across cell membranes (e.g., ion channels, transporters).
- Motor proteins: Generate movement (e.g., myosin, kinesin).
- Hormones: Chemical messengers that regulate various physiological processes (e.g., insulin, growth hormone).
- Antibodies: Part of the immune system, protecting against pathogens.
- Receptor proteins: Bind to specific molecules and trigger cellular responses.
Amino Acid Modifications and Post-Translational Modifications
The 20 standard amino acids are not the only components of proteins. Many proteins undergo post-translational modifications (PTMs) after they are synthesized. These modifications can alter the protein's function, localization, or stability. Examples of PTMs include:
- Phosphorylation: Addition of a phosphate group.
- Glycosylation: Addition of carbohydrate groups.
- Ubiquitination: Addition of ubiquitin, a small protein involved in protein degradation.
Conclusion: The Central Role of Amino Acids in Life
The monomers of proteins, amino acids, are far more than just simple building blocks. Their diverse chemical properties and the intricate way they assemble dictate the incredible complexity and functional versatility of proteins. From the simplest enzyme to the most complex structural protein, amino acids are the fundamental units responsible for the myriad of processes that sustain life. Understanding the structure, properties, and interactions of these monomers is essential for understanding the fundamental mechanisms of life itself. Further research continues to unravel the intricacies of protein folding, modification, and function, leading to advancements in various fields, including medicine, biotechnology, and materials science. The ongoing study of amino acids and their role in protein structure remains a cornerstone of biological investigation, promising further breakthroughs in our understanding of life's fundamental processes.
Latest Posts
Latest Posts
-
Barriers To Entry For Monopolistic Competition
Apr 01, 2025
-
The Most Reactive Metals Are The
Apr 01, 2025
-
How To Find The Perpendicular Vector
Apr 01, 2025
-
What Are The Factors In An Experiment
Apr 01, 2025
-
What Is In The Cranial Cavity
Apr 01, 2025
Related Post
Thank you for visiting our website which covers about The Monomers Of Proteins Are Called . We hope the information provided has been useful to you. Feel free to contact us if you have any questions or need further assistance. See you next time and don't miss to bookmark.