The Voltage Across A Membrane Is Called The _____.
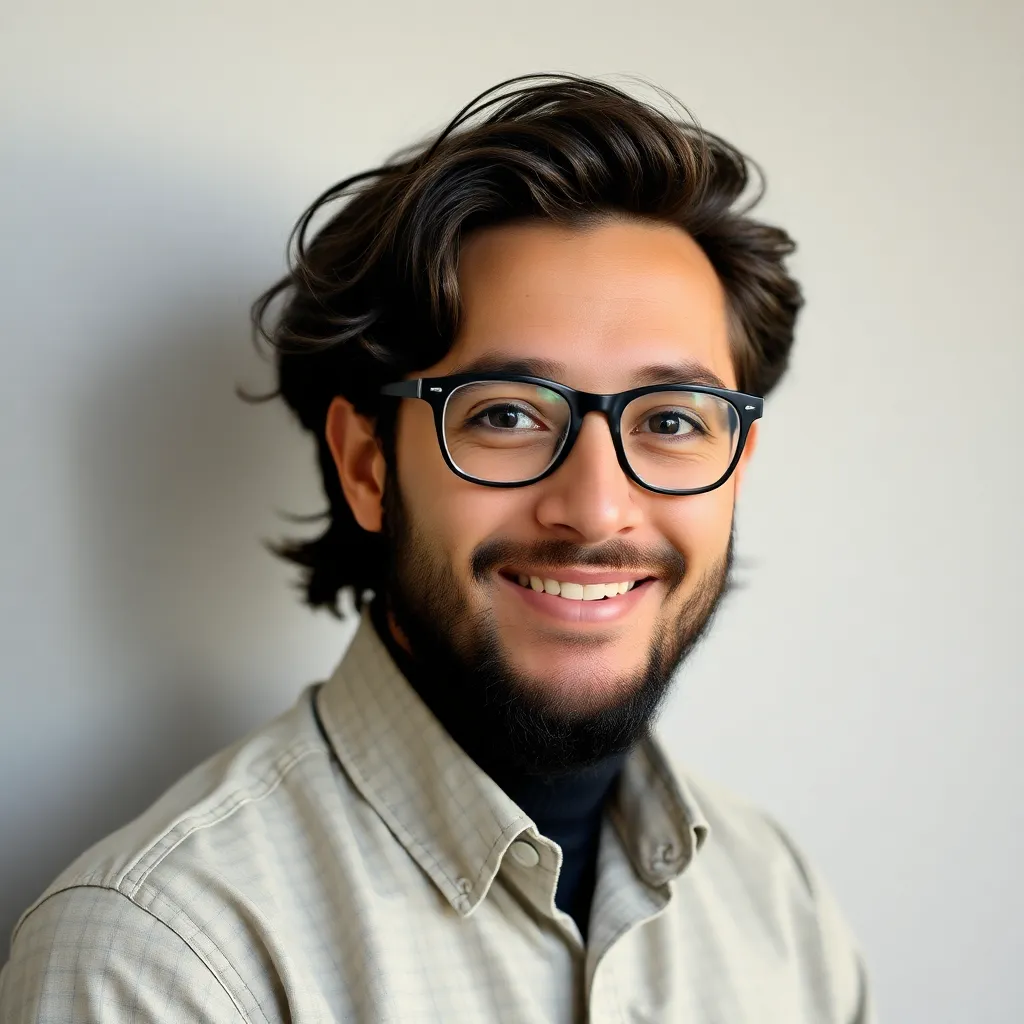
Muz Play
Apr 11, 2025 · 6 min read

Table of Contents
The Voltage Across a Membrane is Called the Membrane Potential
The voltage across a cell membrane, a critical aspect of cellular function, is known as the membrane potential. This electrical potential difference is a fundamental characteristic of all living cells, driving various physiological processes vital for life. Understanding membrane potential is key to comprehending how cells communicate, transport molecules, and maintain homeostasis. This article delves deep into the intricacies of membrane potential, exploring its origins, measurement, significance, and the factors influencing its value.
What is Membrane Potential?
In essence, the membrane potential is the difference in electrical charge between the inside and the outside of a cell. This difference arises from an uneven distribution of ions, primarily potassium (K+), sodium (Na+), chloride (Cl-), and negatively charged proteins, across the cell membrane. The interior of the cell typically carries a negative charge relative to the exterior, a state often described as a polarized membrane. This voltage difference is usually expressed in millivolts (mV) and is usually in the range of -40 mV to -90 mV, depending on the cell type and its physiological state.
The Role of Ion Channels and Pumps
The precise control of ion distribution across the membrane is achieved through specialized membrane proteins: ion channels and pumps.
-
Ion channels: These are protein pores that allow specific ions to passively diffuse across the membrane down their concentration gradients. Selective permeability of these channels plays a critical role in establishing the membrane potential. For instance, potassium leak channels allow a relatively large outward flux of potassium ions, contributing to the negative intracellular charge.
-
Ion pumps: These are active transport proteins that use energy (usually from ATP hydrolysis) to move ions against their concentration gradients. The most prominent example is the sodium-potassium pump (Na+/K+ ATPase). This pump actively extrudes three sodium ions (Na+) out of the cell and brings two potassium ions (K+) into the cell for every ATP molecule hydrolyzed. This pump contributes significantly to the membrane potential by maintaining the concentration gradients of sodium and potassium.
Measuring Membrane Potential: The Patch Clamp Technique
The membrane potential can be accurately measured using a technique called patch clamping. This sophisticated electrophysiological technique involves the use of a glass micropipette with a very fine tip to create a tight seal on a small patch of the cell membrane. By applying a voltage across the micropipette, the membrane potential of the patch can be measured directly. Patch clamping allows researchers to study the activity of individual ion channels and their contribution to the overall membrane potential.
The Resting Membrane Potential: A State of Equilibrium
When a cell is at rest and not actively signaling, it exhibits a relatively stable membrane potential called the resting membrane potential. This potential represents a balance between the passive diffusion of ions through ion channels and the active transport of ions by pumps. While it’s a “resting” potential, it’s not static; it's a dynamic equilibrium constantly maintained by the ongoing activity of ion channels and pumps.
The Goldman-Hodgkin-Katz Equation
The precise value of the resting membrane potential can be predicted using the Goldman-Hodgkin-Katz (GHK) equation. This equation takes into account the permeability of the membrane to different ions and their respective concentration gradients, providing a more accurate representation of the membrane potential than simpler models. It emphasizes the crucial role of relative permeability in determining the resting membrane potential. For example, if the membrane is more permeable to potassium than to sodium, the resting membrane potential will be closer to the potassium equilibrium potential.
The Importance of Membrane Potential
The membrane potential is not merely a static electrical property; it's a dynamic signaling mechanism vital for a multitude of cellular functions:
-
Nerve Impulse Transmission: The propagation of nerve impulses relies heavily on changes in membrane potential. The arrival of a stimulus triggers depolarization – a shift in membrane potential towards a less negative value – that propagates as an action potential along the nerve axon. Repolarization, the restoration of the resting membrane potential, is essential for the nerve to recover and prepare for the next signal.
-
Muscle Contraction: Similar to nerve impulse transmission, muscle contraction is initiated by changes in membrane potential. Depolarization of muscle cells leads to calcium release from intracellular stores, triggering the contractile machinery.
-
Hormone Secretion: Many endocrine cells release hormones in response to changes in membrane potential. Depolarization can trigger the opening of voltage-gated calcium channels, increasing intracellular calcium and leading to exocytosis of hormone-containing vesicles.
-
Nutrient Uptake and Transport: The membrane potential can influence the transport of nutrients and other molecules across the cell membrane. For example, the electrochemical gradient established by the membrane potential can drive the uptake of certain ions and nutrients.
-
Cell Signaling: Changes in membrane potential can serve as signals between cells. For example, in some types of synapses, the release of neurotransmitters is triggered by changes in the presynaptic membrane potential.
Factors Affecting Membrane Potential
Several factors can influence the value of the membrane potential:
-
Ion Concentrations: Changes in the intracellular or extracellular concentrations of ions like sodium, potassium, and chloride will directly affect the membrane potential. Disruptions to these gradients can lead to significant changes in cellular function.
-
Ion Channel Activity: The opening or closing of ion channels dramatically alters the membrane permeability to different ions, causing shifts in membrane potential. Various stimuli can influence ion channel activity, including neurotransmitters, hormones, and changes in membrane voltage.
-
Temperature: Temperature changes can alter the activity of ion channels and pumps, influencing the membrane potential.
-
pH: Changes in extracellular pH can affect the activity of ion channels and the distribution of ions, thereby altering the membrane potential.
-
Drugs and Toxins: Many drugs and toxins can target ion channels and pumps, disrupting membrane potential and leading to various physiological effects.
Membrane Potential and Diseases
Disruptions to the membrane potential can have severe consequences and contribute to a variety of diseases:
-
Cardiac Arrhythmias: Abnormal changes in the membrane potential of cardiac cells can lead to irregular heartbeats, a condition known as arrhythmia. This can range from mild palpitations to life-threatening conditions.
-
Neurological Disorders: Many neurological disorders are associated with disruptions in neuronal membrane potential. Examples include epilepsy, characterized by excessive neuronal excitability, and certain types of paralysis caused by impaired nerve impulse transmission.
-
Muscle Disorders: Disorders affecting muscle membrane potential can result in muscle weakness or paralysis. Conditions like myasthenia gravis involve impaired neuromuscular transmission.
Conclusion: A Dynamic Equilibrium Essential for Life
The membrane potential is far more than just a voltage across a cell membrane. It's a highly dynamic and finely regulated process critical for the functioning of all living cells. The intricate interplay between ion channels, pumps, and ion concentration gradients carefully maintains this potential, shaping cellular communication, signal transduction, and overall cellular homeostasis. Understanding the intricacies of membrane potential is crucial not only for basic biological research but also for developing effective treatments for a range of human diseases where disruptions to this essential process play a significant role. Further research in this field will undoubtedly continue to shed light on the complexities and importance of this fundamental cellular property.
Latest Posts
Latest Posts
-
Chemistry A Molecular Approach Nivaldo J Tro
Apr 18, 2025
-
Difference Between Equilibrium And Steady State
Apr 18, 2025
-
Part B The Replication Fork
Apr 18, 2025
-
When Thermal Energy Is Removed From Particles What Action Occurs
Apr 18, 2025
-
Atomic Mass Is Equivalent To The Number Of
Apr 18, 2025
Related Post
Thank you for visiting our website which covers about The Voltage Across A Membrane Is Called The _____. . We hope the information provided has been useful to you. Feel free to contact us if you have any questions or need further assistance. See you next time and don't miss to bookmark.