Understanding Periodic Trends In Atomic Ionizability
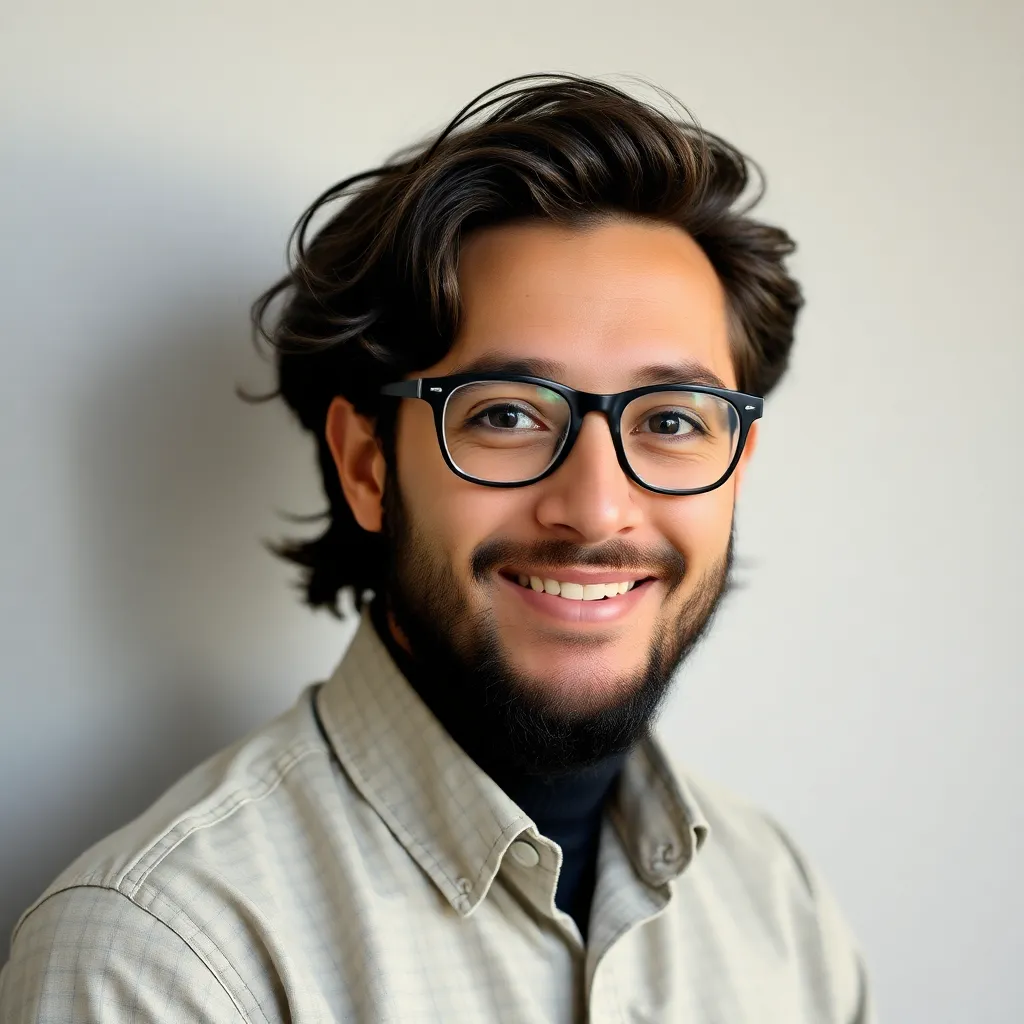
Muz Play
Apr 16, 2025 · 6 min read

Table of Contents
Understanding Periodic Trends in Atomic Ionizability
Ionization energy, the minimum energy required to remove an electron from a neutral gaseous atom, is a fundamental property reflecting an atom's stability and reactivity. Understanding periodic trends in atomic ionizability is crucial for predicting chemical behavior and explaining the properties of elements and their compounds. This comprehensive guide delves into the factors influencing ionization energy, explores the periodic trends, and examines exceptions to these trends.
Factors Affecting Ionization Energy
Several key factors interplay to determine an atom's ionization energy:
1. Effective Nuclear Charge (Z<sub>eff</sub>):
The effective nuclear charge represents the net positive charge experienced by the outermost electrons. A higher Z<sub>eff</sub> implies a stronger attraction between the nucleus and valence electrons, leading to a higher ionization energy. This is because inner electrons shield the outer electrons from the full positive charge of the nucleus, reducing the effective nuclear charge experienced by the valence electrons. Shielding is more effective with more inner electrons.
2. Atomic Radius:
As atomic radius increases, the distance between the nucleus and the valence electrons increases. This larger distance weakens the electrostatic attraction between the nucleus and the valence electrons, resulting in a lower ionization energy. Larger atoms generally have lower ionization energies.
3. Electron Shielding:
Electron shielding, as mentioned earlier, is the effect of inner electrons reducing the attractive force of the nucleus on the outer electrons. Electrons in the same shell experience less shielding from each other than from electrons in inner shells. Increased shielding decreases the effective nuclear charge and thus the ionization energy.
4. Electron Configuration:
The electron configuration of an atom significantly influences its ionization energy. Elements with a half-filled or fully filled subshell (e.g., p<sup>3</sup> or p<sup>6</sup>) exhibit higher ionization energies than those with partially filled subshells. This extra stability associated with half-filled or fully filled subshells requires more energy to remove an electron.
5. Penetration Effect:
Different orbitals within the same shell do not have the same probability density near the nucleus. Electrons in s orbitals penetrate closer to the nucleus than electrons in p orbitals, experiencing a stronger nuclear attraction. This penetration effect means s electrons are more tightly bound and require more energy to remove than p electrons in the same shell. Similarly, p electrons are more tightly bound than d electrons, and d electrons are more tightly bound than f electrons within the same principal energy level.
Periodic Trends in Ionization Energy
The periodic table beautifully organizes elements according to their properties, and ionization energy exhibits clear periodic trends:
1. Trend Across a Period (Left to Right):
Moving across a period from left to right, the atomic radius generally decreases, and the effective nuclear charge increases. Both contribute to a significant increase in ionization energy. The added protons attract the outer electrons more strongly, making it increasingly difficult to remove an electron. This trend is particularly evident across periods where the shielding effect remains relatively constant while the nuclear charge increases.
2. Trend Down a Group (Top to Bottom):
Moving down a group, the atomic radius significantly increases due to the addition of electron shells. The increased distance between the nucleus and the valence electrons weakens the electrostatic attraction, leading to a decrease in ionization energy. The shielding effect of inner electrons also plays a crucial role, further reducing the effective nuclear charge experienced by the outermost electrons.
3. First, Second, and Subsequent Ionization Energies:
The first ionization energy refers to the energy required to remove the first electron. Subsequent ionization energies (second, third, etc.) increase significantly because removing an electron from a positively charged ion requires overcoming the stronger electrostatic attraction between the more positively charged ion and the remaining electrons. The increase in ionization energy between successive ionizations is generally substantial. For example, the second ionization energy of an alkali metal is substantially larger than its first ionization energy. This is because removing an electron from a positive ion (cation) requires substantially more energy compared to removing an electron from a neutral atom.
Exceptions to the General Trends:
While the general trends in ionization energy are well-established, some exceptions exist:
1. Boron and Aluminum:
Boron (B) and aluminum (Al) exhibit slightly lower ionization energies than their preceding elements (Be and Mg). This is because removing an electron from a p orbital (2p in B and 3p in Al) is easier than removing an electron from a filled s orbital (2s in Be and 3s in Mg) in the same principal energy level. The p orbital electrons are less tightly held due to less effective penetration.
2. Oxygen and Nitrogen:
Nitrogen (N) has a higher first ionization energy than oxygen (O), despite oxygen having a higher effective nuclear charge. This anomaly arises due to the stability of nitrogen's half-filled p subshell (p<sup>3</sup> configuration). Removing an electron from this stable configuration requires more energy than removing an electron from oxygen's partially filled p subshell (p<sup>4</sup> configuration), even though oxygen has a slightly higher effective nuclear charge.
3. Other Exceptions:
Other deviations from the general trends can occur due to complex interactions between effective nuclear charge, electron shielding, and electron-electron repulsion. Careful consideration of these factors is necessary for interpreting the ionization energies of specific elements.
Applications and Importance of Understanding Ionization Energy:
Understanding ionization energy has far-reaching implications in various fields:
-
Predicting Chemical Reactivity: Elements with low ionization energies tend to be more reactive because they readily lose electrons to form cations. Elements with high ionization energies tend to be less reactive because they strongly hold onto their electrons.
-
Spectroscopy: Ionization energy is directly related to the energy levels of electrons within an atom. Spectroscopic techniques can measure ionization energies, providing insights into atomic structure and electron configurations.
-
Material Science: Ionization energy is crucial in understanding the behavior of materials. For example, the ionization energy of dopant atoms determines their effectiveness in semiconductor materials.
-
Astrophysics: Ionization energies are essential for understanding stellar atmospheres and the composition of interstellar gas. Spectral analysis of starlight reveals the ionization states of atoms, providing clues to the temperature and density of the stars.
-
Chemistry and Chemical Bonding: Ionization energy plays a critical role in determining the type of chemical bonds formed between atoms. It helps in explaining the formation of ionic and covalent bonds.
Conclusion:
Ionization energy is a fundamental property governing the reactivity and behavior of elements. The periodic trends in ionization energy are largely determined by the interplay of effective nuclear charge, atomic radius, electron shielding, electron configuration, and penetration effects. While general trends are established, exceptions exist, often due to the inherent complexities of electron-electron interactions and orbital configurations. Understanding these trends and exceptions is essential for comprehending the chemical properties of elements and their application in diverse fields. Further exploration into the nuances of electron configuration and subtle interactions influencing effective nuclear charge enhances our understanding of this critical atomic property.
Latest Posts
Latest Posts
-
The Krebs Cycle Takes Place In
Apr 19, 2025
-
Confidence Interval For Slope Of Regression Line Formula
Apr 19, 2025
-
What Is The Difference Between Culture And Society
Apr 19, 2025
-
During His Trip On The Beagle Darwin Found That
Apr 19, 2025
-
Explain The Structure And Function Of The Respiratory Membrane
Apr 19, 2025
Related Post
Thank you for visiting our website which covers about Understanding Periodic Trends In Atomic Ionizability . We hope the information provided has been useful to you. Feel free to contact us if you have any questions or need further assistance. See you next time and don't miss to bookmark.