What Holds Atoms Together In Compounds
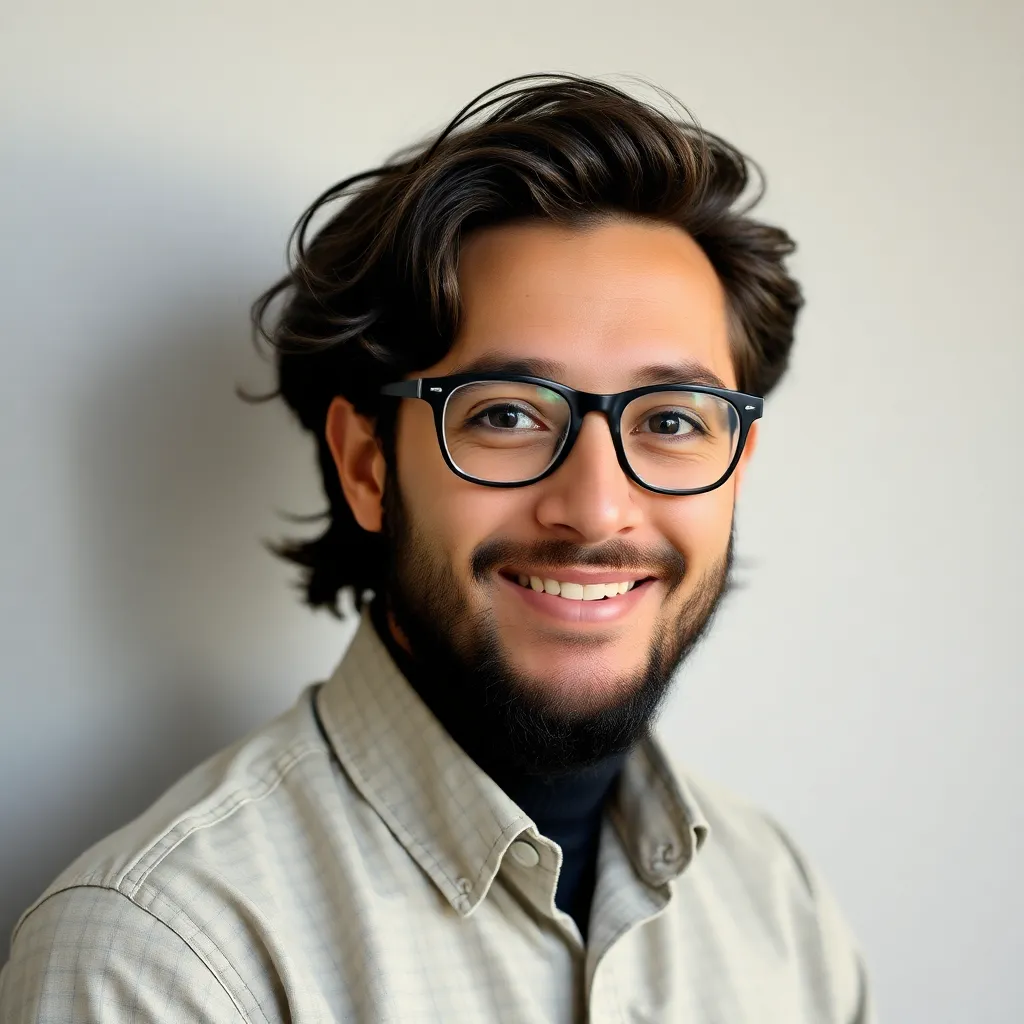
Muz Play
May 10, 2025 · 7 min read
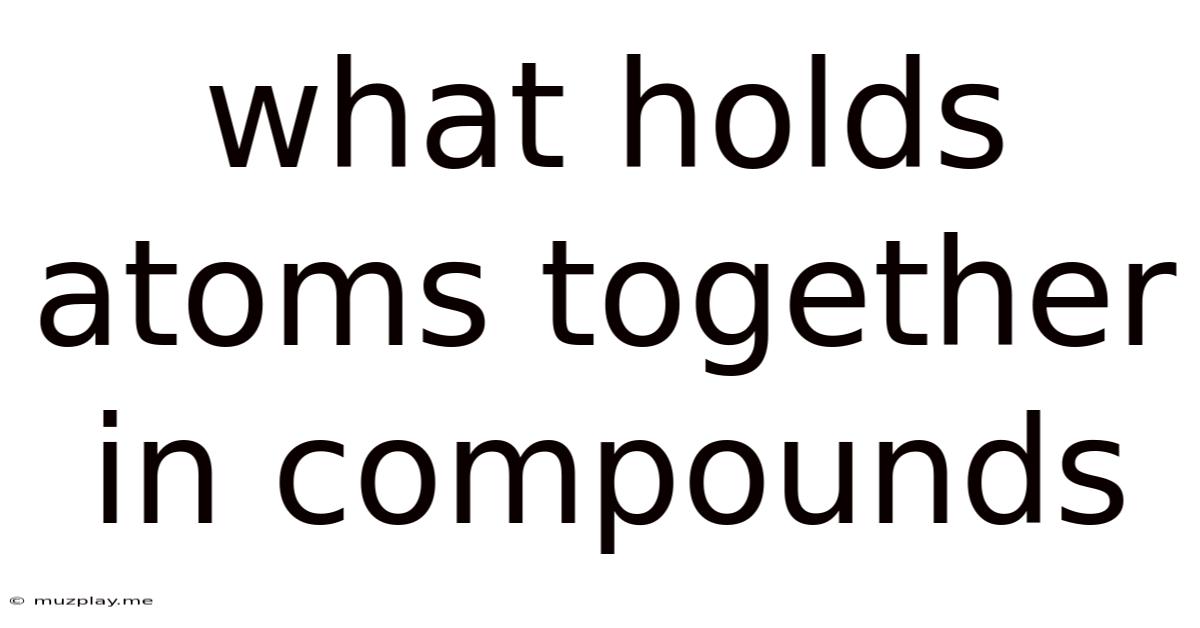
Table of Contents
What Holds Atoms Together in Compounds? A Deep Dive into Chemical Bonding
Understanding how atoms combine to form the diverse array of compounds that make up our world is fundamental to chemistry. This isn't simply atoms sticking together; it's a complex interplay of fundamental forces that dictate the properties and behaviors of all matter. This article will delve into the fascinating world of chemical bonding, exploring the primary mechanisms – ionic bonding, covalent bonding, and metallic bonding – that govern the formation of compounds. We'll also touch upon weaker intermolecular forces that influence the macroscopic properties of substances.
The Driving Force: Achieving Stability
Atoms are inherently driven towards stability. This stability is typically achieved by having a full outer electron shell, often referred to as a valence shell. This configuration, often resembling that of a noble gas, minimizes the atom's energy. Atoms achieve this stability through the sharing or transfer of electrons, leading to the formation of chemical bonds.
1. Ionic Bonding: The Electrostatic Attraction
Ionic bonding is characterized by the electrostatic attraction between oppositely charged ions. This type of bonding occurs between atoms with significantly different electronegativities – a measure of an atom's ability to attract electrons in a chemical bond. Highly electronegative atoms, such as those in Group 17 (halogens), tend to gain electrons, becoming negatively charged ions called anions. Conversely, atoms with low electronegativity, such as those in Group 1 (alkali metals), tend to lose electrons, becoming positively charged ions called cations.
How it works: The strong electrostatic force of attraction between the positively charged cation and the negatively charged anion forms the ionic bond. This bond is relatively strong, resulting in compounds with high melting and boiling points and often forming crystalline structures. The transfer of electrons is complete, resulting in distinct ions within the crystal lattice.
Examples: Sodium chloride (NaCl), commonly known as table salt, is a classic example of an ionic compound. Sodium (Na), an alkali metal, readily loses one electron to achieve a stable electron configuration, forming the Na⁺ cation. Chlorine (Cl), a halogen, readily gains one electron to achieve a stable electron configuration, forming the Cl⁻ anion. The electrostatic attraction between the Na⁺ and Cl⁻ ions forms the ionic bond, creating the crystal lattice structure of NaCl. Other examples include magnesium oxide (MgO), potassium iodide (KI), and calcium fluoride (CaF₂).
Factors influencing ionic bond strength: The strength of an ionic bond is influenced by several factors, including the charge of the ions and the distance between them. Higher charges lead to stronger attraction, while larger distances weaken the attraction. The size of the ions also plays a role; smaller ions lead to stronger bonds due to closer proximity.
2. Covalent Bonding: Sharing is Caring
In contrast to ionic bonding, covalent bonding involves the sharing of electrons between atoms. This type of bonding typically occurs between atoms with similar electronegativities, often nonmetals. Instead of a complete transfer of electrons, atoms share electrons to achieve a stable electron configuration.
How it works: Atoms share one or more pairs of electrons, forming a covalent bond. The shared electrons are attracted to the nuclei of both atoms, holding them together. The shared electron pair occupies a region of space between the two nuclei, forming a molecular orbital.
Types of covalent bonds: Covalent bonds can be single, double, or triple bonds, depending on the number of electron pairs shared. Single bonds involve one shared electron pair, double bonds involve two shared electron pairs, and triple bonds involve three shared electron pairs. The number of bonds influences the bond length and strength; triple bonds are shorter and stronger than double bonds, which are shorter and stronger than single bonds.
Polar and Nonpolar Covalent Bonds: The electronegativity difference between the atoms involved in a covalent bond determines the polarity of the bond. If the electronegativity difference is small (typically less than 0.5), the bond is considered nonpolar, meaning the electrons are shared equally between the atoms. If the electronegativity difference is significant (typically greater than 0.5), the bond is considered polar, meaning the electrons are shared unequally, resulting in a partial positive charge (δ⁺) on one atom and a partial negative charge (δ⁻) on the other.
Examples: Water (H₂O) is a classic example of a molecule with polar covalent bonds. Oxygen is more electronegative than hydrogen, so the shared electrons are drawn closer to the oxygen atom, creating a partial negative charge on the oxygen and partial positive charges on the hydrogens. Methane (CH₄) is an example of a molecule with nonpolar covalent bonds because the electronegativity difference between carbon and hydrogen is very small. Other examples of covalent compounds include carbon dioxide (CO₂), ammonia (NH₃), and ethanol (C₂H₅OH).
3. Metallic Bonding: A Sea of Electrons
Metallic bonding is a type of bonding found in metals. It is characterized by the delocalization of valence electrons throughout a lattice of metal atoms. Unlike ionic and covalent bonding, where electrons are localized between specific atoms, in metallic bonding, the valence electrons are free to move throughout the entire metal structure.
How it works: The metal atoms lose their valence electrons, forming positive ions. These electrons are not associated with any particular atom but rather form a "sea" of delocalized electrons that surrounds the metal cations. The electrostatic attraction between the positively charged metal ions and the sea of delocalized electrons holds the metal together.
Properties resulting from metallic bonding: The delocalized electrons are responsible for many of the characteristic properties of metals, such as their high electrical and thermal conductivity, malleability (ability to be hammered into sheets), and ductility (ability to be drawn into wires). The ease with which electrons can move accounts for their conductivity. The "sea" of electrons also allows metal atoms to slide past each other without disrupting the metallic bonds, explaining their malleability and ductility.
Examples: Iron (Fe), copper (Cu), gold (Au), and aluminum (Al) are all examples of metals with metallic bonding. The strength and properties of metallic bonds vary depending on the metal and its crystal structure.
Weaker Intermolecular Forces: Shaping Macroscopic Properties
While ionic, covalent, and metallic bonds are the primary forces holding atoms together within molecules or crystals, weaker intermolecular forces also play crucial roles in determining the macroscopic properties of substances. These forces act between molecules, not within them.
Types of Intermolecular Forces: These include:
-
London Dispersion Forces (LDFs): These are the weakest intermolecular forces and are present in all molecules. They arise from temporary fluctuations in electron distribution, creating temporary dipoles that induce dipoles in neighboring molecules. The strength of LDFs increases with the size and shape of the molecule.
-
Dipole-Dipole Forces: These forces occur between polar molecules, where permanent dipoles attract each other. The partially positive end of one molecule attracts the partially negative end of another.
-
Hydrogen Bonding: This is a special type of dipole-dipole force that occurs when a hydrogen atom bonded to a highly electronegative atom (such as oxygen, nitrogen, or fluorine) is attracted to another highly electronegative atom in a nearby molecule. Hydrogen bonding is relatively strong compared to other intermolecular forces and plays a vital role in the properties of water and many biological molecules.
Conclusion: A Complex Interplay of Forces
The formation of compounds is a fascinating interplay of fundamental forces. Ionic, covalent, and metallic bonding are the primary mechanisms that hold atoms together, each with unique characteristics influenced by the electronegativity and electron configuration of the atoms involved. Weaker intermolecular forces further shape the macroscopic properties of substances, demonstrating the intricate complexity of chemical interactions that govern the world around us. Understanding these bonding principles is crucial to comprehending the behavior and properties of all matter, from the simplest molecules to the most complex materials. Further exploration into specific bonding types and their applications in various fields will reveal even more intricacies of this captivating subject.
Latest Posts
Latest Posts
-
Find The Class With The Least Number Of Data Values
May 10, 2025
-
What Is The Relationship Between Proportion And Scale
May 10, 2025
-
How Many Protons And Neutrons Are In Cl 37
May 10, 2025
-
Region Of Dna That Recruits The Transcriptional Machinery
May 10, 2025
-
Low Sun Angles Result In Reduced Solar Energy Because
May 10, 2025
Related Post
Thank you for visiting our website which covers about What Holds Atoms Together In Compounds . We hope the information provided has been useful to you. Feel free to contact us if you have any questions or need further assistance. See you next time and don't miss to bookmark.