What Is Reversible And Irreversible Process In Thermodynamics
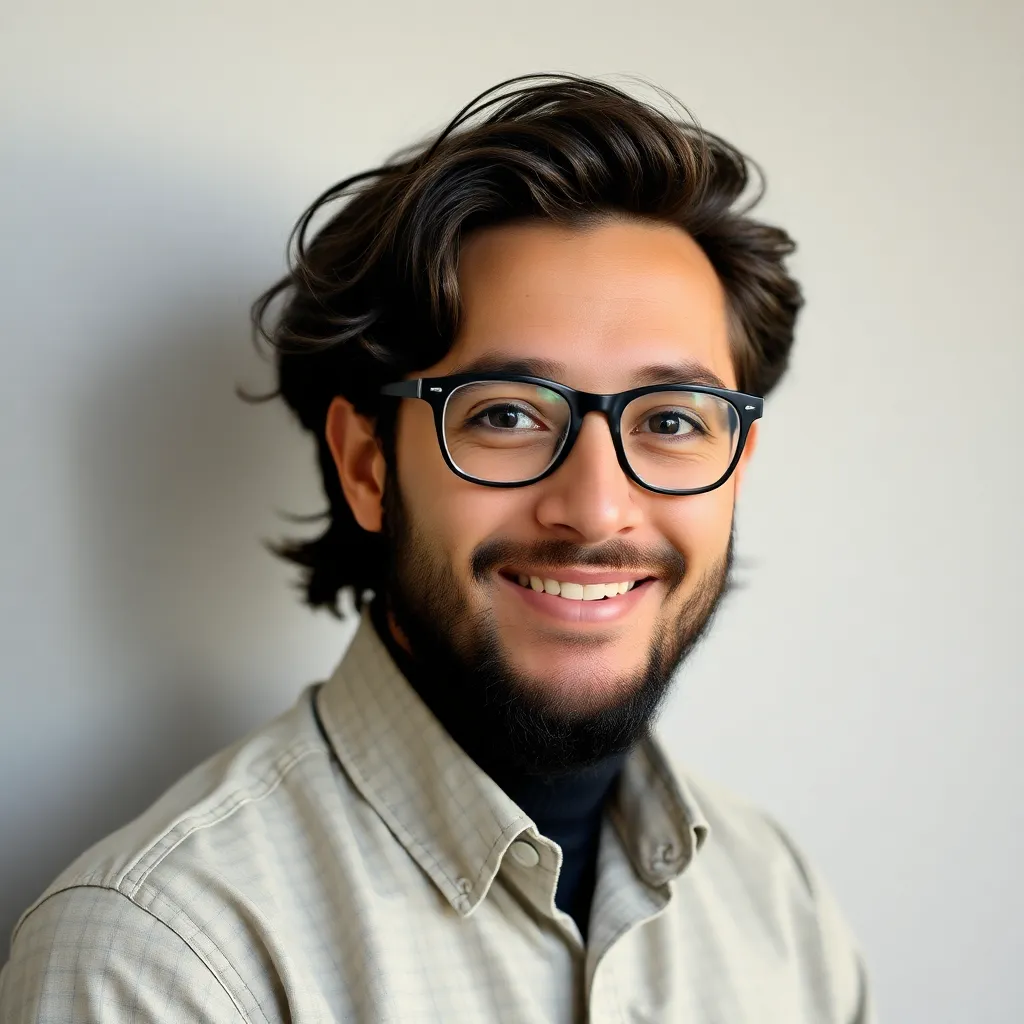
Muz Play
Mar 31, 2025 · 6 min read
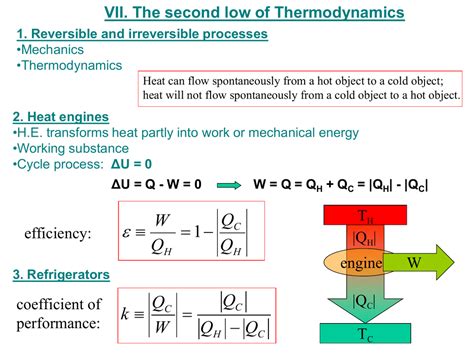
Table of Contents
What is Reversible and Irreversible Process in Thermodynamics?
Thermodynamics, the study of heat and its relation to energy and work, relies heavily on the concepts of reversible and irreversible processes. Understanding the distinction between these two types of processes is crucial for grasping fundamental thermodynamic principles and applying them to various systems. This article delves deep into the definitions, characteristics, examples, and implications of reversible and irreversible processes, offering a comprehensive understanding of this vital aspect of thermodynamics.
Defining Reversible Processes
A reversible process in thermodynamics is an idealized process that can be reversed without leaving any trace on the surroundings. This means that if the process is reversed, both the system and its surroundings return to their initial states, as if the process never occurred. Crucially, throughout the entire reversible process, the system is always infinitesimally close to equilibrium.
Key Characteristics of Reversible Processes:
- Infinitesimally Slow: A reversible process occurs infinitely slowly, allowing the system to remain in equilibrium at each step. Any deviation from equilibrium would introduce irreversibility.
- No Friction or Dissipative Forces: There are no frictional forces or other dissipative forces present that would cause energy loss. This ensures that the system's energy is conserved perfectly during the process and its reversal.
- Isothermal or Adiabatic Changes: Reversible processes can be isothermal (constant temperature) or adiabatic (no heat exchange), depending on the specific conditions. However, they must always be carried out in a controlled manner to maintain equilibrium.
- Equilibrium Throughout: The system remains in equilibrium with its surroundings throughout the reversible process. This ensures that the changes are gradual and reversible.
- Theoretical Idealization: It's important to note that perfectly reversible processes are purely theoretical. Real-world processes always involve some degree of irreversibility due to factors like friction, heat loss, and finite reaction rates.
Defining Irreversible Processes
In contrast to reversible processes, an irreversible process is any process that cannot be reversed without leaving some change in the system or its surroundings. These changes typically manifest as an increase in entropy within the combined system and surroundings.
Key Characteristics of Irreversible Processes:
- Finite Rate: Irreversible processes occur at a finite rate. The system is not always in equilibrium throughout the process.
- Presence of Friction or Dissipative Forces: Friction, viscosity, and other dissipative forces are present, causing energy loss in the form of heat.
- Spontaneous Changes: Many natural processes are inherently irreversible, driven by a tendency towards increased entropy (disorder). Examples include heat transfer from hot to cold objects, free expansion of gases, and chemical reactions.
- Uncontrolled Conditions: Irreversible processes often occur under uncontrolled conditions, leading to deviations from equilibrium and energy dissipation.
- Entropy Increase: The hallmark of an irreversible process is an increase in the total entropy of the system and surroundings. This increase is always positive (ΔS > 0).
Examples of Reversible and Irreversible Processes
Let's illustrate the differences with concrete examples:
Reversible Process Examples (Idealized):
- Isothermal Expansion of an Ideal Gas: Imagine a piston slowly moving outwards against a very small opposing force. The gas expands slowly and isothermally (constant temperature), maintaining equilibrium throughout the process. If the process is reversed by slowly pushing the piston inwards, the gas will return to its original state, with no net change in the system or surroundings.
- Reversible Isothermal Compression: The inverse of the above. The gas is compressed very slowly and isothermally, again maintaining equilibrium at all times. Reversing the process results in a return to the initial state.
- Reversible Adiabatic Expansion/Compression: A similar process to the isothermal versions, but now without heat exchange with the surroundings. The temperature of the system changes during the expansion or compression, but the process remains reversible due to the infinitely slow nature and maintenance of equilibrium.
Irreversible Process Examples:
- Free Expansion of a Gas: If a gas is allowed to expand freely into a vacuum, the process is irreversible. The gas expands rapidly and chaotically, without maintaining equilibrium. Reversing this process requires external work and will not fully restore the initial state.
- Heat Transfer Between Bodies at Different Temperatures: When heat flows from a hot body to a colder body, it’s an irreversible process. Spontaneously, heat moves from high to low temperatures, and it's impossible to reverse this without putting in additional work, resulting in a net change to the surroundings.
- Chemical Reactions: Most chemical reactions are irreversible under ordinary conditions. Once reactants are converted into products, reversing the reaction generally requires a significant input of energy or a change in reaction conditions.
- Mixing of Gases: Mixing two different gases is an irreversible process. While you can physically separate the gases, this requires work and won't fully undo the changes made to the system's entropy.
- Friction: Any process involving friction is irreversible. The energy lost due to friction is converted to heat, and this heat cannot be completely recovered to restore the initial state.
- Electrical Resistance: The flow of current through a resistor is an irreversible process. Electrical energy is transformed into heat, and this transformation cannot be fully reversed.
The Role of Entropy in Reversible and Irreversible Processes
Entropy (S) is a crucial thermodynamic property representing the disorder or randomness of a system. The second law of thermodynamics states that the total entropy of an isolated system can only increase over time, or remain constant in ideal cases where the system is in a steady state or undergoing a reversible process.
-
Reversible Processes and Entropy: In a reversible process, the change in entropy of the system is equal and opposite to the change in entropy of the surroundings. Therefore, the total entropy change (ΔS_total) for a reversible process is zero: ΔS_total = ΔS_system + ΔS_surroundings = 0.
-
Irreversible Processes and Entropy: In contrast, irreversible processes always lead to a net increase in the total entropy of the universe. The entropy of the surroundings might decrease, but the increase in the system's entropy will always be larger, resulting in a positive ΔS_total > 0. This increase in entropy reflects the inherent irreversibility of the process and its tendency to dissipate energy.
Practical Implications and Applications
Understanding reversible and irreversible processes is critical for numerous applications in:
- Engineering Design: Engineers strive to minimize irreversibilities in designs to improve efficiency. For instance, reducing friction in engines, using more efficient heat exchangers, and employing better insulation contribute to increased efficiency.
- Chemical Engineering: Chemical processes are often analyzed using thermodynamic principles to understand efficiency and equilibrium. Identifying and minimizing irreversible steps is key to optimizing reaction yields and minimizing energy consumption.
- Power Generation: Thermodynamic cycles, such as the Rankine cycle used in power plants, are analyzed to determine their efficiency. Identifying irreversible components allows engineers to design more effective power generation systems.
- Climate Science: Understanding irreversible processes, such as the melting of polar ice caps, is vital for assessing the impact of climate change.
Conclusion
The distinction between reversible and irreversible processes forms a cornerstone of thermodynamics. While reversible processes are theoretical ideals, understanding them provides a framework for analyzing real-world processes, many of which are inherently irreversible. By recognizing the role of entropy, dissipative forces, and the inherent tendency toward disorder, we can better understand and optimize a wide range of systems and processes in various fields of science and engineering. The irreversible nature of most real-world phenomena underscores the second law of thermodynamics and its pervasive influence on the universe. Further exploration of these concepts continues to be a vital area of research and development.
Latest Posts
Latest Posts
-
What Is The Chemical Composition Of Amethyst
Apr 01, 2025
-
Clonal Selection Of T Cells Happens In The Thymus
Apr 01, 2025
-
Formula Para Sacar Diametro De Un Circulo
Apr 01, 2025
-
How To Make A Frequency Histogram In Excel
Apr 01, 2025
-
What Is The Monomer Of A Dna Molecule
Apr 01, 2025
Related Post
Thank you for visiting our website which covers about What Is Reversible And Irreversible Process In Thermodynamics . We hope the information provided has been useful to you. Feel free to contact us if you have any questions or need further assistance. See you next time and don't miss to bookmark.