What Is The Final Electron Acceptor In Anaerobic Respiration
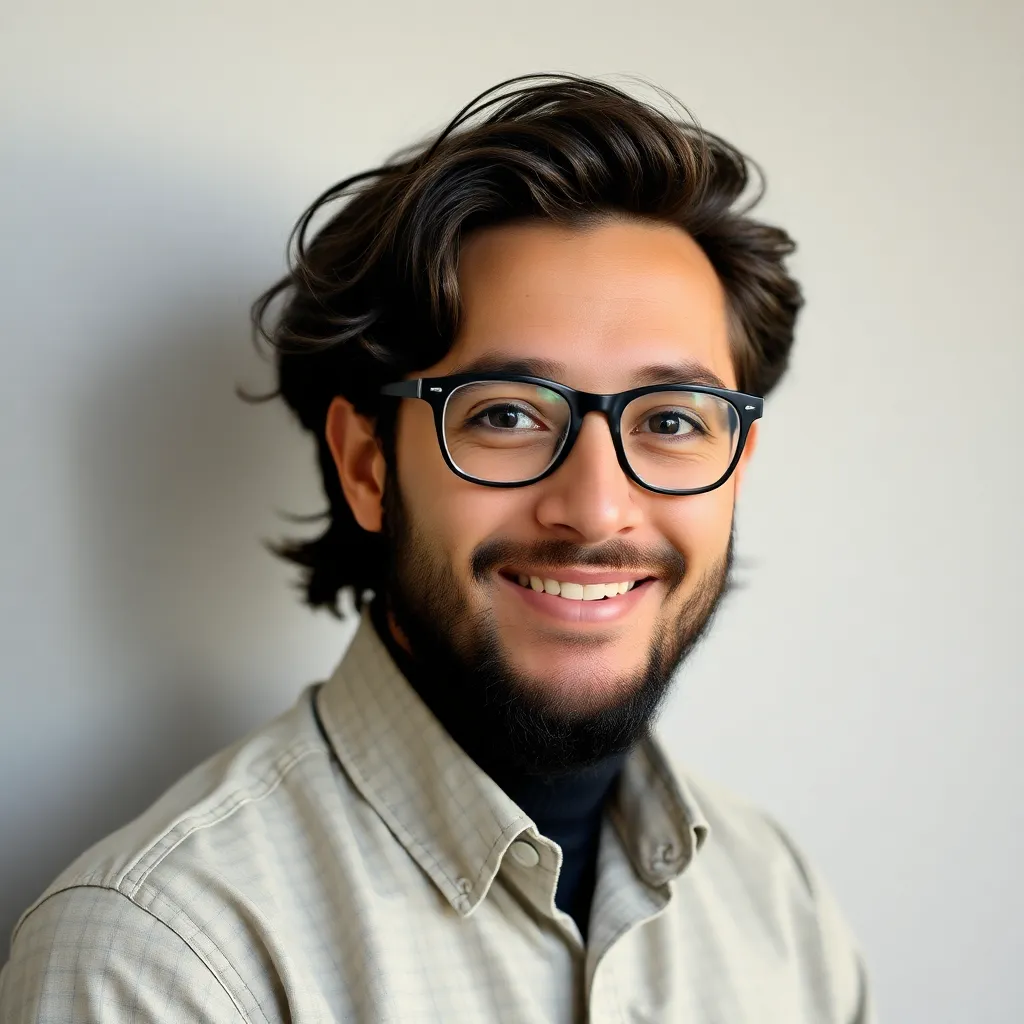
Muz Play
Mar 28, 2025 · 6 min read
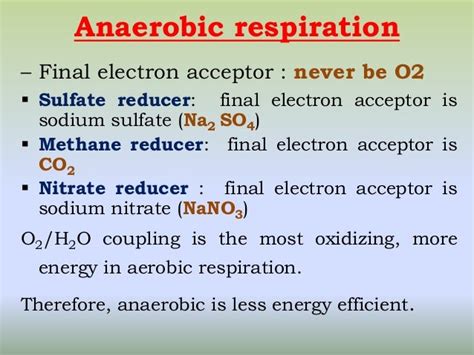
Table of Contents
What is the Final Electron Acceptor in Anaerobic Respiration?
Anaerobic respiration, unlike its aerobic counterpart, doesn't utilize oxygen as the final electron acceptor in the electron transport chain. This fundamental difference significantly impacts the energy yield and the types of organisms capable of employing this metabolic pathway. Understanding the various final electron acceptors in anaerobic respiration is crucial to appreciating the diverse strategies life has evolved to thrive in oxygen-depleted environments. This comprehensive exploration delves into the mechanisms, energetics, and ecological significance of anaerobic respiration, clarifying the diverse molecules that serve as the terminal electron sinks.
The Electron Transport Chain: A Universal Energy Harvesting System
Before discussing the specific final electron acceptors, it's essential to understand the underlying principle: the electron transport chain (ETC). Both aerobic and anaerobic respiration utilize an ETC, a series of redox reactions where electrons are passed from electron donors (like NADH and FADH2) to electron acceptors of progressively higher reduction potential. This electron flow releases energy, which is used to pump protons (H+) across a membrane, creating a proton gradient. This gradient then drives ATP synthesis through chemiosmosis, the process powering the majority of cellular functions.
The crucial difference lies in the final electron acceptor. In aerobic respiration, this is oxygen (O2), a highly electronegative molecule that readily accepts electrons. In anaerobic respiration, however, oxygen is absent, and the cell must rely on alternative electron acceptors. These acceptors are typically less electronegative than oxygen, resulting in a smaller free energy change and, consequently, a lower ATP yield compared to aerobic respiration.
Diverse Final Electron Acceptors: A Spectrum of Anaerobic Strategies
The variety of anaerobic respiration strategies reflects the remarkable adaptability of life. Different organisms have evolved to utilize different inorganic molecules as final electron acceptors, depending on their environmental niche and the availability of these molecules. Let's explore some of the most common:
1. Nitrate (NO3-) Reduction: The Nitrate Respiration
Nitrate reduction, also known as denitrification, is a widespread anaerobic respiratory process. Bacteria employing this strategy use nitrate (NO3-) as the terminal electron acceptor. The process involves a series of enzymatic reductions, converting nitrate to nitrite (NO2-), nitric oxide (NO), nitrous oxide (N2O), and finally, dinitrogen gas (N2).
Key Aspects of Nitrate Respiration:
- Enzyme involvement: Nitrate reductases catalyze the initial reduction steps, while nitrite reductases, nitric oxide reductases, and nitrous oxide reductases handle the subsequent steps.
- Ecological significance: Denitrification plays a crucial role in the nitrogen cycle, converting biologically available nitrogen (nitrate) into gaseous forms (N2O and N2), which are released into the atmosphere. This process contributes to greenhouse gas emissions and can impact air quality.
- Energy yield: The energy yield from nitrate reduction is lower than that from oxygen respiration, but it's sufficient for the survival of many bacterial species in oxygen-deprived environments.
2. Sulfate (SO42-) Reduction: The Sulfate Respiration
Sulfate-reducing bacteria (SRB) utilize sulfate (SO42-) as their final electron acceptor. This process is significant in anaerobic environments rich in sulfate, such as marine sediments and swamps. The reduction of sulfate to sulfide (S2-) is a multi-step process involving several intermediate compounds.
Key Aspects of Sulfate Respiration:
- ATP production: Similar to other anaerobic respiration pathways, sulfate reduction generates ATP via chemiosmosis, albeit with a lower efficiency compared to aerobic respiration.
- Environmental impact: The production of hydrogen sulfide (H2S) by SRB is a significant environmental factor. H2S is toxic to many organisms and contributes to the characteristic smell of swamps and marshes.
- Industrial applications: Certain aspects of sulfate reduction are exploited in bioremediation strategies, using SRB to treat sulfate-contaminated wastewaters.
3. Carbon Dioxide (CO2) Reduction: Methanogenesis and Acetogenesis
Methanogens, a unique group of archaea, use carbon dioxide (CO2) as the final electron acceptor, reducing it to methane (CH4). This process, called methanogenesis, is crucial in anaerobic environments such as wetlands, rice paddies, and the digestive tracts of ruminant animals.
Key Aspects of Methanogenesis:
- Unique biochemistry: Methanogenesis involves unique coenzymes and metabolic pathways distinct from those found in bacteria.
- Environmental significance: Methane is a potent greenhouse gas, and methanogens play a crucial role in the global carbon cycle.
- Industrial applications: Methane produced through methanogenesis can be harnessed as a biofuel source.
Acetogenesis, another CO2 reduction pathway, leads to acetate (CH3COO-) production instead of methane. This process is less efficient in terms of energy conservation than methanogenesis.
4. Ferric Iron (Fe3+) Reduction: A Significant Pathway
Iron-reducing bacteria utilize ferric iron (Fe3+), a prevalent form of iron in many environments, as the final electron acceptor, reducing it to ferrous iron (Fe2+). This process is particularly important in iron-rich soils and sediments.
Key Aspects of Iron Reduction:
- Electron transfer mechanisms: Iron reduction can involve direct electron transfer to Fe3+ or indirect transfer through electron shuttles.
- Environmental significance: Iron reduction significantly impacts iron cycling in various ecosystems.
- Bioremediation applications: Iron-reducing bacteria are being investigated for their potential in bioremediation of contaminated sites, reducing the toxicity of certain pollutants.
5. Other Inorganic Electron Acceptors
Beyond the aforementioned acceptors, other inorganic molecules can serve as terminal electron acceptors in anaerobic respiration, including:
- Manganese (Mn4+): Manganese-reducing bacteria convert manganese oxides (Mn4+) to soluble manganese (Mn2+).
- Urinary tract infection causing agents: Some bacteria causing urinary tract infections use fumarate in the process of anaerobic respiration.
- Selenate (SeO42-): Selenate-reducing bacteria transform selenate to less toxic forms of selenium.
- Arsenate (AsO43-): Arsenate-reducing bacteria convert arsenate to arsenite, a process of environmental concern.
Energetics of Anaerobic Respiration: Lower Yield, but Essential
The energy yield of anaerobic respiration is considerably lower than that of aerobic respiration. This is because the reduction potentials of alternative electron acceptors are lower than that of oxygen. Consequently, the proton motive force generated during anaerobic respiration is weaker, leading to less ATP production.
However, it's important to emphasize that while the yield is lower, anaerobic respiration provides essential energy for survival in oxygen-deprived environments. Many organisms have adapted to thrive in these conditions, relying on the energy generated through anaerobic respiration to sustain their metabolism.
Ecological Significance: Shaping Life in Oxygen-Free Niches
Anaerobic respiration is not a niche process; it's a fundamental metabolic strategy shaping life on Earth. It underpins the functioning of various ecosystems, including:
- Marine sediments: Sulfate reduction and methanogenesis are major processes in deep-sea sediments, impacting nutrient cycling and ecosystem structure.
- Soil environments: Nitrate reduction and iron reduction significantly influence nutrient availability and soil fertility.
- Animal digestive tracts: Methanogenesis in the guts of ruminants contributes to methane emissions and has implications for agriculture.
- Wastewater treatment: Anaerobic respiration is exploited in wastewater treatment to break down organic matter and reduce pollution.
Conclusion: A Metabolic Tapestry of Adaptation
Anaerobic respiration encompasses a diverse array of metabolic strategies, each adapted to specific environmental conditions and electron acceptor availability. While the energy yield is lower than that of aerobic respiration, these processes are essential for sustaining life in oxygen-deprived environments. Understanding the mechanisms, energetics, and ecological significance of anaerobic respiration is crucial for appreciating the remarkable adaptability of life and the intricate interplay between organisms and their environment. Further research continues to uncover new aspects of anaerobic respiration, revealing even greater diversity and complexity in this crucial metabolic pathway. The continuing investigation into the intricacies of anaerobic respiration holds implications for various fields including biotechnology, bioremediation, and climate change research.
Latest Posts
Latest Posts
-
To Find The Total Resistance In A Series Circuit
Mar 31, 2025
-
Root And Shoot System Of A Plant
Mar 31, 2025
-
Dna Coloring Transcription And Translation
Mar 31, 2025
-
If Mean Is Greater Than Median
Mar 31, 2025
-
Equation Of Charging And Discharging Of Capacitor
Mar 31, 2025
Related Post
Thank you for visiting our website which covers about What Is The Final Electron Acceptor In Anaerobic Respiration . We hope the information provided has been useful to you. Feel free to contact us if you have any questions or need further assistance. See you next time and don't miss to bookmark.