Why Is Energy Needed For Active Transport
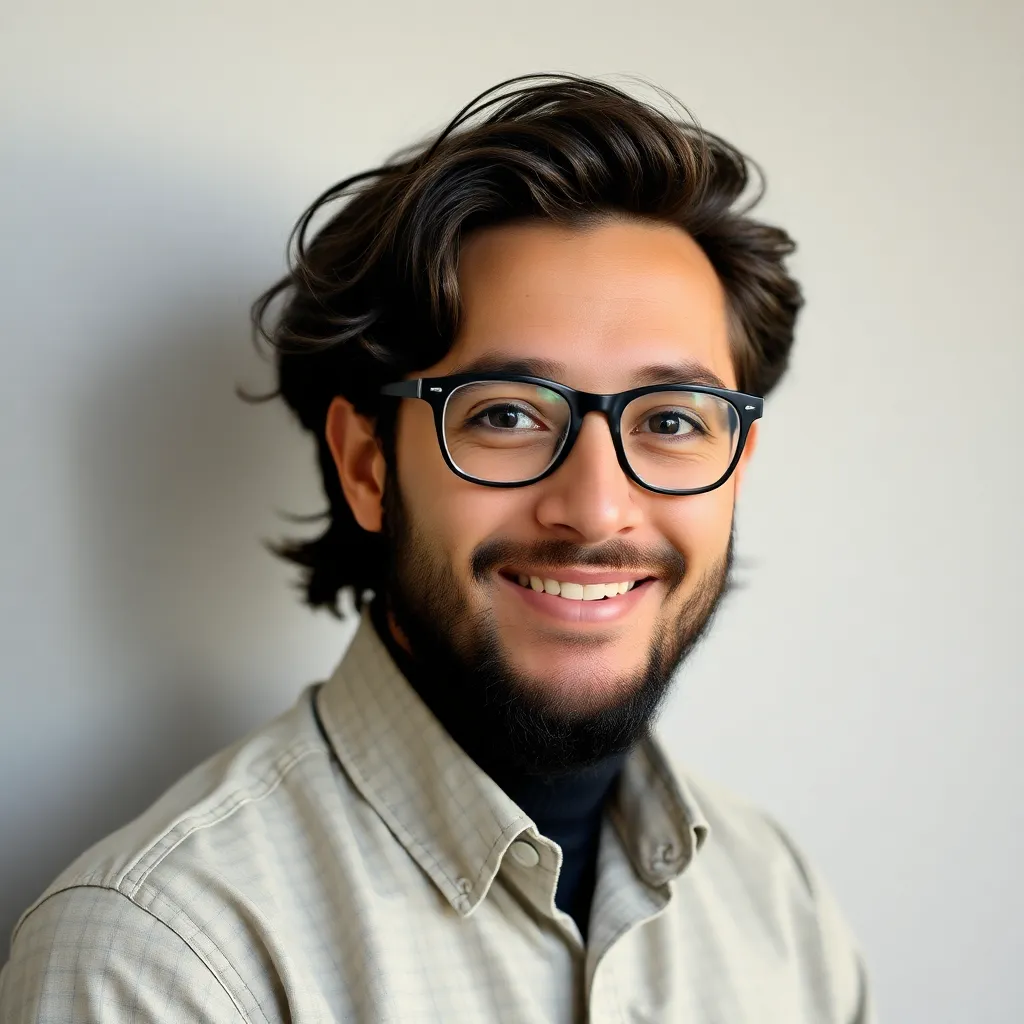
Muz Play
Apr 23, 2025 · 6 min read

Table of Contents
Why is Energy Needed for Active Transport?
Active transport, a fundamental process in biology, is the movement of molecules across a cell membrane against their concentration gradient. This means moving molecules from an area of low concentration to an area of high concentration, a process that seemingly defies the natural tendency of things to move from high to low concentration (passive transport). This uphill battle requires energy input, making it distinct from passive transport mechanisms. Understanding why energy is needed for active transport is crucial to grasping the intricacies of cellular function and homeostasis.
The Energetic Cost of Defying Equilibrium
The core reason energy is required for active transport lies in the second law of thermodynamics. This law states that the total entropy (disorder) of an isolated system can only increase over time. Moving molecules against their concentration gradient creates a more ordered state – a higher concentration in one area. This decrease in entropy is thermodynamically unfavorable; it requires an input of energy to overcome this inherent resistance and push the system away from equilibrium.
Think of it like rolling a ball uphill. Naturally, the ball will roll downhill, towards a state of lower potential energy. To move the ball uphill, you must exert energy to overcome gravity. Similarly, active transport must expend energy to overcome the natural tendency of molecules to diffuse down their concentration gradient.
The Role of ATP: The Cell's Energy Currency
The primary energy source fueling most active transport processes is adenosine triphosphate (ATP). ATP is a high-energy molecule that acts as the cell's energy currency. Hydrolysis of ATP, the breaking down of ATP into adenosine diphosphate (ADP) and inorganic phosphate (Pi), releases a significant amount of energy that can be harnessed to power various cellular activities, including active transport.
The energy released during ATP hydrolysis is often used to directly or indirectly drive the conformational changes in transport proteins, enabling them to bind to and translocate molecules against their concentration gradient. This process is analogous to using energy to change the shape of the "ball-rolling machine," allowing it to move the ball uphill.
Types of Active Transport and Their Energy Requirements
Active transport mechanisms can be broadly categorized into two main types:
1. Primary Active Transport: Direct ATP Hydrolysis
In primary active transport, the energy derived from ATP hydrolysis is directly coupled to the movement of molecules. The transport protein itself is an enzyme, often called an ATPase, that hydrolyzes ATP and uses the released energy to change its conformation, thereby transporting the molecule across the membrane.
A prime example is the sodium-potassium pump (Na+/K+ ATPase). This ubiquitous pump maintains the electrochemical gradients of sodium (Na+) and potassium (K+) ions across the cell membrane, a process essential for nerve impulse transmission, muscle contraction, and many other cellular functions. It uses the energy from ATP hydrolysis to move three Na+ ions out of the cell and two K+ ions into the cell, against their respective concentration gradients.
Other examples of primary active transport include:
- Proton pumps (H+ ATPases): These pumps move protons (H+) across membranes, creating a proton gradient that is often used to drive other transport processes or generate ATP (in chemiosmosis). They are crucial in maintaining cellular pH and powering processes like oxidative phosphorylation.
- Calcium pumps (Ca2+ ATPases): These pumps actively remove calcium ions from the cytoplasm, maintaining low cytosolic calcium concentrations that are crucial for regulating various cellular processes.
2. Secondary Active Transport: Indirect ATP Hydrolysis
Secondary active transport utilizes the energy stored in an electrochemical gradient, often created by primary active transport, to drive the movement of other molecules. It doesn't directly hydrolyze ATP; instead, it leverages the energy stored in the pre-existing gradient.
This type of transport often involves co-transporters or symporters, which move two different molecules in the same direction, and antiporters or exchangers, which move two different molecules in opposite directions. One molecule moves down its concentration gradient (releasing energy), providing the driving force for the other molecule to move against its concentration gradient.
A classic example is the sodium-glucose co-transporter (SGLT1) in the intestines and kidneys. The movement of sodium ions down their concentration gradient (established by the Na+/K+ pump) provides the energy to transport glucose against its concentration gradient into the cells.
Other examples include:
- Sodium-amino acid co-transporters: These transporters use the sodium gradient to move amino acids into cells.
- Chloride-bicarbonate exchanger: This antiporter exchanges chloride and bicarbonate ions across the cell membrane, playing a crucial role in maintaining blood pH.
The Importance of Active Transport in Biological Systems
Active transport is indispensable for maintaining cellular homeostasis and facilitating various vital functions. Its role extends across a wide range of biological processes:
- Maintaining ion gradients: Active transport is crucial for establishing and maintaining the electrochemical gradients of ions like Na+, K+, Ca2+, and H+ across cell membranes. These gradients are vital for nerve impulse transmission, muscle contraction, and many other cellular processes.
- Nutrient uptake: Cells rely on active transport to absorb essential nutrients like glucose, amino acids, and vitamins against their concentration gradients from the extracellular environment.
- Waste removal: Active transport mechanisms remove metabolic waste products from cells, preventing their accumulation and potential toxicity.
- Signal transduction: Active transport plays a critical role in signal transduction pathways by regulating the intracellular concentration of signaling molecules.
- Endocytosis and Exocytosis: These processes, which involve the bulk transport of materials into and out of cells, often depend on active transport mechanisms to power vesicle movement and membrane fusion.
Consequences of Active Transport Dysfunction
Malfunctions in active transport systems can lead to a range of severe physiological consequences. For example:
- Cystic fibrosis: This genetic disorder involves a defect in the cystic fibrosis transmembrane conductance regulator (CFTR), a chloride ion channel crucial for fluid secretion. The malfunctioning CFTR leads to thick mucus buildup in the lungs and other organs.
- Cardiac arrhythmias: Dysfunctions in the Na+/K+ pump can disrupt the electrochemical gradients crucial for proper heart rhythm, leading to cardiac arrhythmias.
- Muscle weakness: Disruptions in calcium homeostasis, due to problems with calcium pumps, can lead to muscle weakness and fatigue.
- Neurological disorders: Impaired active transport can significantly affect nerve function, contributing to various neurological disorders.
Conclusion: Active Transport - A Necessary Energy Investment
Active transport, despite its energy expenditure, is a crucial process for life. It allows cells to maintain internal environments distinct from their surroundings, enabling them to perform their specialized functions effectively. The energy investment in active transport is ultimately a necessary cost for maintaining cellular order, homeostasis, and the overall survival of the organism. Understanding the intricacies of active transport and its energy requirements provides a deeper understanding of the complex machinery that sustains life at the cellular level. Further research continues to unravel the precise mechanisms and regulatory aspects of active transport, revealing more about its fundamental role in biological systems and offering potential avenues for therapeutic interventions in various diseases.
Latest Posts
Latest Posts
-
Cost Of Goods Manufactured Journal Entry
Apr 23, 2025
-
An Atoms Mass Number Equals The Number Of
Apr 23, 2025
-
Did Women Fight In The Colosseum
Apr 23, 2025
-
A Covalent Bond Involves The Sharing Of
Apr 23, 2025
-
Compare And Contrast Pure Research And Applied Research
Apr 23, 2025
Related Post
Thank you for visiting our website which covers about Why Is Energy Needed For Active Transport . We hope the information provided has been useful to you. Feel free to contact us if you have any questions or need further assistance. See you next time and don't miss to bookmark.