Does Secondary Active Transport Require Atp
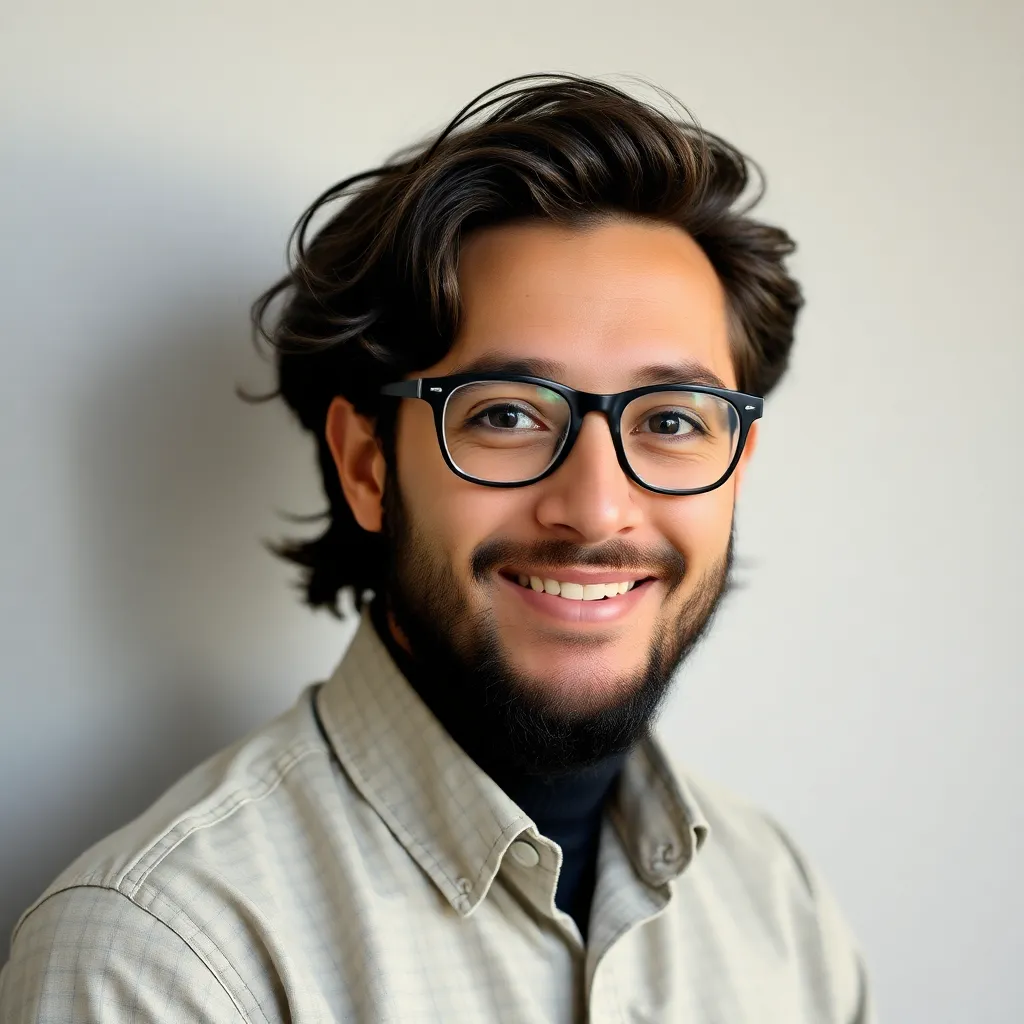
Muz Play
Apr 03, 2025 · 5 min read

Table of Contents
Does Secondary Active Transport Require ATP? A Deep Dive into Co-transport Mechanisms
Secondary active transport, a crucial process in cell biology, often sparks confusion regarding its energy dependence. While it doesn't directly utilize ATP like primary active transport, it's fundamentally reliant on the energy stored within electrochemical gradients created by primary active transport. This article will delve into the intricacies of secondary active transport, clarifying its relationship with ATP and exploring the various mechanisms involved.
Understanding the Fundamentals: Primary vs. Secondary Active Transport
Before examining the specifics of secondary active transport, it's essential to understand the distinction between it and primary active transport. This contrast highlights the indirect ATP dependence of secondary active transport.
Primary active transport directly utilizes the energy released from ATP hydrolysis to move molecules against their concentration gradient. Examples include the sodium-potassium pump (Na+/K+ ATPase), which maintains the electrochemical gradient across cell membranes, and the calcium pump (Ca2+ ATPase), crucial for regulating intracellular calcium levels. These pumps are directly powered by ATP.
Secondary active transport, on the other hand, leverages the energy stored in an electrochemical gradient established by primary active transport. This gradient, usually involving ions like sodium (Na+), provides the driving force for the movement of other molecules. Crucially, secondary active transport doesn't directly hydrolyze ATP; its energy source is the pre-existing gradient created by a primary active transporter that did use ATP.
The Key Role of Electrochemical Gradients
The electrochemical gradient is the key player enabling secondary active transport. This gradient is a combination of two factors:
- Chemical gradient: The difference in concentration of a molecule across a membrane. Molecules tend to move from areas of high concentration to areas of low concentration.
- Electrical gradient: The difference in electrical charge across a membrane. Positively charged ions are attracted to negatively charged areas, and vice versa.
The combined effect of these gradients creates a driving force capable of moving molecules against their own concentration gradients. This is the energy source harnessed by secondary active transport.
Mechanisms of Secondary Active Transport: Symport and Antiport
Secondary active transport operates through two primary mechanisms: symport and antiport.
1. Symport (Co-transport): In symport, the movement of one molecule down its electrochemical gradient (the driving ion) provides the energy to transport another molecule against its concentration gradient in the same direction. The classic example is the sodium-glucose co-transporter (SGLT1), found in the intestines and kidneys. The influx of sodium ions (Na+), driven by the sodium gradient maintained by the Na+/K+ ATPase, drags glucose molecules into the cell against their concentration gradient. Note: While glucose moves against its gradient, the sodium gradient is the actual energy source; it's the primary active transport that created this gradient which originally used ATP.
2. Antiport (Exchange): Antiport involves the movement of one molecule down its electrochemical gradient in one direction, providing the energy to transport another molecule against its gradient in the opposite direction. A prominent example is the sodium-calcium exchanger (NCX), which removes calcium ions from the cell. The influx of sodium ions, driven by the sodium gradient, fuels the extrusion of calcium ions against their concentration gradient. Again, the original source of energy for this is the ATP-dependent primary active transport that established the sodium gradient.
Indirect ATP Dependence: A Crucial Clarification
It's crucial to reiterate that secondary active transport does not directly consume ATP. However, it's indirectly dependent on ATP because the electrochemical gradient it utilizes is generated by primary active transport systems, which directly hydrolyze ATP. Without the functioning Na+/K+ ATPase, for example, the sodium gradient driving many secondary active transport systems would collapse, rendering them ineffective.
Think of it like this: primary active transport is like charging a battery (using ATP). Secondary active transport then uses the energy stored in that charged battery (the electrochemical gradient) to perform its work. The battery needs recharging (by primary active transport utilizing ATP), but the device running off the battery (secondary active transport) does not directly use the energy source for charging.
Examples of Secondary Active Transport in Biological Systems
Secondary active transport plays critical roles in various physiological processes:
- Nutrient absorption in the intestines: The SGLT1 co-transporter absorbs glucose and other nutrients from the intestinal lumen into the bloodstream.
- Reabsorption of substances in the kidneys: Secondary active transport mechanisms reabsorb essential molecules like glucose, amino acids, and ions from the kidney filtrate back into the bloodstream.
- Regulation of intracellular calcium levels: The NCX removes calcium from cells, maintaining appropriate calcium concentrations for muscle contraction, nerve impulse transmission, and other cellular processes.
- Neurotransmitter reuptake: Specific transporters utilize sodium gradients to remove neurotransmitters from the synaptic cleft, terminating neuronal signaling.
Consequences of Dysfunction in Secondary Active Transport
Disruptions in secondary active transport systems can lead to severe physiological consequences. For instance:
- Genetic defects in SGLT1 can cause glucose-galactose malabsorption, leading to diarrhea and dehydration.
- Impaired renal reabsorption of glucose, due to defects in secondary active transport, results in glucosuria (glucose in the urine).
- Dysregulation of intracellular calcium, a consequence of impaired NCX function, can contribute to various diseases, including cardiac arrhythmias and muscle disorders.
Conclusion: A Complex Interplay of Energy and Transport
Secondary active transport is a sophisticated and vital mechanism enabling cells to move molecules against their concentration gradients without directly consuming ATP. It’s crucial to remember its indirect dependence on the electrochemical gradients established by ATP-driven primary active transport. This elegant interplay highlights the complexity and efficiency of cellular processes, where energy is carefully managed and utilized for optimal functionality. Understanding the nuances of both primary and secondary active transport is essential for appreciating the intricate workings of living cells and the potential implications of their dysfunction in various diseases. Further research continuously reveals the profound impact of these transport mechanisms on cellular homeostasis and overall health.
Latest Posts
Latest Posts
-
Which Of The Following May Use Rna As Its Genome
Apr 04, 2025
-
Impact Of Lincolns Assassination On Reconstruction
Apr 04, 2025
-
The Sampling Distribution Of The Difference Helps Us Determine
Apr 04, 2025
-
Exterior Angles Of A Circle Theorem
Apr 04, 2025
-
Why Is Soil Considered A Non Renewable Resource
Apr 04, 2025
Related Post
Thank you for visiting our website which covers about Does Secondary Active Transport Require Atp . We hope the information provided has been useful to you. Feel free to contact us if you have any questions or need further assistance. See you next time and don't miss to bookmark.