Proteins Are Made Up Of Monomers Called
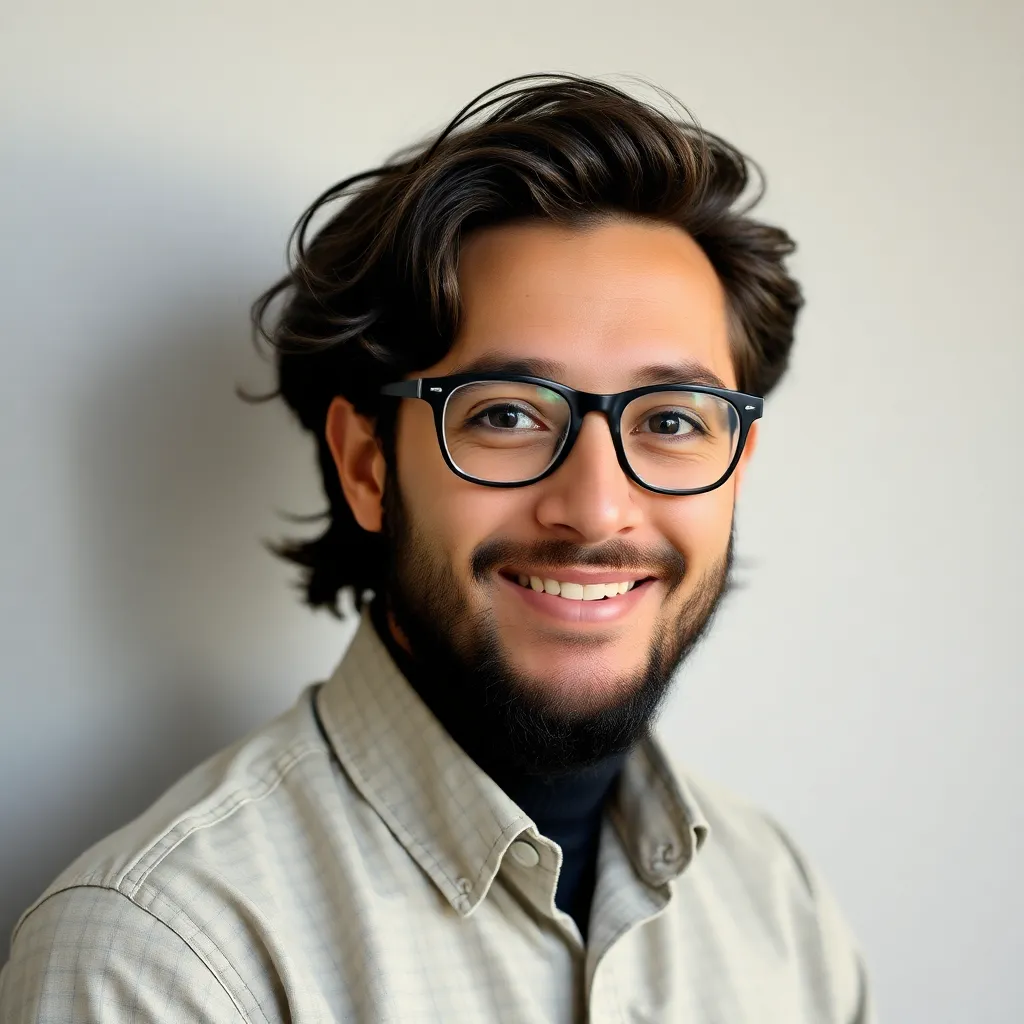
Muz Play
Mar 26, 2025 · 7 min read
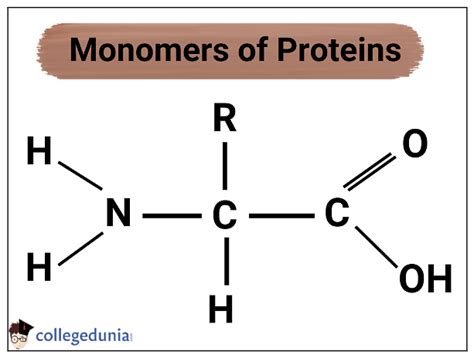
Table of Contents
Proteins Are Made Up of Monomers Called Amino Acids: A Deep Dive
Proteins are the workhorses of the cell, carrying out a vast array of functions crucial for life. From catalyzing biochemical reactions as enzymes to providing structural support as components of connective tissues, proteins are essential for virtually every biological process. But what are these remarkable molecules actually made of? The answer lies in their fundamental building blocks: amino acids.
Understanding the Monomer: Amino Acids
Proteins are polymers, meaning they are large molecules composed of repeating smaller units called monomers. In the case of proteins, these monomers are amino acids. There are 20 standard amino acids that make up the proteins found in all living organisms. While variations exist, these 20 form the core alphabet of protein construction.
Each amino acid shares a common basic structure:
- A central carbon atom (α-carbon): This carbon atom is bonded to four different groups.
- An amino group (-NH2): This is a basic group, meaning it can accept a proton (H+), and contributes to the amino acid's overall charge.
- A carboxyl group (-COOH): This is an acidic group, meaning it can donate a proton (H+), and also influences the amino acid's charge.
- A hydrogen atom (H): This is a simple hydrogen atom.
- A variable side chain (R-group): This is the unique part of each amino acid and is what differentiates one amino acid from another. The R-group varies in size, shape, charge, and polarity, and it dictates the amino acid's chemical properties and how it interacts with other molecules.
The Diversity of Amino Acid Side Chains
The R-group is what gives each amino acid its unique characteristics. These variations lead to a remarkable diversity in protein structure and function:
-
Nonpolar, aliphatic R-groups: These R-groups are hydrophobic (water-fearing) and are often found buried within the protein's core, away from the aqueous environment of the cell. Examples include glycine, alanine, valine, leucine, isoleucine, and methionine.
-
Aromatic R-groups: These R-groups contain aromatic rings, making them relatively hydrophobic. Examples include phenylalanine, tyrosine, and tryptophan.
-
Polar, uncharged R-groups: These R-groups are hydrophilic (water-loving) and are often found on the protein's surface, interacting with the surrounding water molecules. Examples include serine, threonine, cysteine, asparagine, and glutamine.
-
Positively charged (basic) R-groups: These R-groups carry a positive charge at physiological pH and are strongly hydrophilic. Examples include lysine, arginine, and histidine.
-
Negatively charged (acidic) R-groups: These R-groups carry a negative charge at physiological pH and are also strongly hydrophilic. Examples include aspartic acid and glutamic acid.
The unique properties of each amino acid's side chain influence its interactions with other amino acids within a protein and ultimately determine the protein's overall three-dimensional structure and function. This interaction is crucial for protein folding and stability.
From Amino Acids to Proteins: Peptide Bonds and Protein Synthesis
Amino acids link together to form proteins through a process called peptide bond formation. This is a dehydration reaction, where a water molecule is removed as the carboxyl group of one amino acid reacts with the amino group of another. The resulting bond between the two amino acids is a peptide bond.
A chain of amino acids linked by peptide bonds is called a polypeptide. Proteins can be composed of one or more polypeptide chains, which can fold into complex three-dimensional structures. The sequence of amino acids in a polypeptide chain, known as the primary structure, dictates how the protein folds and ultimately its function.
Protein Synthesis: The Central Dogma
The process of protein synthesis is elegantly orchestrated by the cell's machinery, following the central dogma of molecular biology: DNA → RNA → Protein. DNA contains the genetic code, which determines the amino acid sequence of a protein. This code is transcribed into messenger RNA (mRNA), which is then translated into a polypeptide chain by ribosomes.
Transcription: The DNA sequence of a gene is copied into a complementary mRNA molecule.
Translation: The mRNA molecule travels to the ribosome, where its sequence is read in codons (three-nucleotide sequences). Each codon specifies a particular amino acid. Transfer RNA (tRNA) molecules, carrying specific amino acids, recognize the codons and deliver the corresponding amino acids to the ribosome, where they are linked together to form the polypeptide chain.
This intricate process ensures the accurate synthesis of proteins, vital for the cell's functioning.
Protein Structure: A Hierarchy of Organization
The three-dimensional structure of a protein is crucial for its function. Protein structure is often described in terms of four levels of organization:
1. Primary Structure: The Amino Acid Sequence
The primary structure of a protein refers to the linear sequence of amino acids in a polypeptide chain. This sequence is determined by the genetic code and is crucial for determining the higher levels of protein structure. Even a single amino acid substitution can drastically alter a protein's function, as famously demonstrated by the sickle cell anemia mutation.
2. Secondary Structure: Local Folding Patterns
Secondary structure refers to local folding patterns within a polypeptide chain, stabilized by hydrogen bonds between the backbone atoms of the amino acids. Common secondary structure elements include:
-
α-helices: A right-handed coiled structure stabilized by hydrogen bonds between the carbonyl oxygen of one amino acid and the amide hydrogen of an amino acid four residues further down the chain.
-
β-sheets: Formed by hydrogen bonds between extended stretches of polypeptide chains arranged side-by-side. β-sheets can be parallel (chains running in the same direction) or antiparallel (chains running in opposite directions).
-
Loops and turns: Regions connecting α-helices and β-sheets. These are often flexible and can be crucial for protein function.
3. Tertiary Structure: The Three-Dimensional Arrangement
Tertiary structure refers to the overall three-dimensional arrangement of a polypeptide chain, including all its secondary structure elements. This structure is stabilized by a variety of interactions, including:
-
Hydrophobic interactions: Nonpolar side chains tend to cluster together in the protein's interior, away from the aqueous environment.
-
Hydrogen bonds: Hydrogen bonds between polar side chains contribute to the protein's stability.
-
Ionic bonds (salt bridges): Interactions between oppositely charged side chains.
-
Disulfide bonds: Covalent bonds between cysteine residues, forming strong cross-links within the protein.
The tertiary structure determines the protein's overall shape and is crucial for its function.
4. Quaternary Structure: Multiple Polypeptide Chains
Some proteins are composed of multiple polypeptide chains, each with its own tertiary structure. The arrangement of these individual chains is referred to as the quaternary structure. These subunits can interact through the same types of interactions that stabilize tertiary structure. Examples of proteins with quaternary structure include hemoglobin and many enzymes.
Protein Function: A Diverse Repertoire
The diverse array of amino acids and the complexity of protein structure enable proteins to perform an incredibly wide range of functions:
-
Enzymes: Catalyze biochemical reactions, accelerating the rate of reactions without being consumed themselves.
-
Structural proteins: Provide support and shape to cells and tissues, such as collagen in connective tissue and keratin in hair and nails.
-
Transport proteins: Carry molecules across cell membranes, such as hemoglobin carrying oxygen in the blood.
-
Motor proteins: Generate movement, such as myosin in muscle cells.
-
Hormones: Chemical messengers that regulate various physiological processes.
-
Receptors: Bind to specific molecules, initiating cellular responses.
-
Antibodies: Part of the immune system, recognizing and neutralizing foreign substances.
Conclusion: The Importance of Amino Acid Sequence and Protein Structure
Proteins are remarkable molecules, crucial for life. Their functions stem directly from the precise arrangement of their constituent amino acids and the resulting three-dimensional structure. Understanding the relationship between amino acid sequence, protein structure, and function is fundamental to understanding how living organisms work and to developing new therapies for diseases caused by protein malfunction. The twenty standard amino acids, each with its unique properties, act as a versatile alphabet, composing the intricate and diverse structures that underpin life's processes. Further research into the intricacies of protein structure and function promises to unveil even more about the complexity and elegance of biological systems.
Latest Posts
Latest Posts
-
According To The Bronsted Lowry Definition
Mar 29, 2025
-
What Are The Vertical Columns Called On A Periodic Table
Mar 29, 2025
-
Where Does Fermentation Take Place In A Cell
Mar 29, 2025
-
What Does A High Specific Heat Mean
Mar 29, 2025
-
Example Of Liquid Dissolved In Liquid
Mar 29, 2025
Related Post
Thank you for visiting our website which covers about Proteins Are Made Up Of Monomers Called . We hope the information provided has been useful to you. Feel free to contact us if you have any questions or need further assistance. See you next time and don't miss to bookmark.