The Flow Of Genetic Information In A Cell Goes From
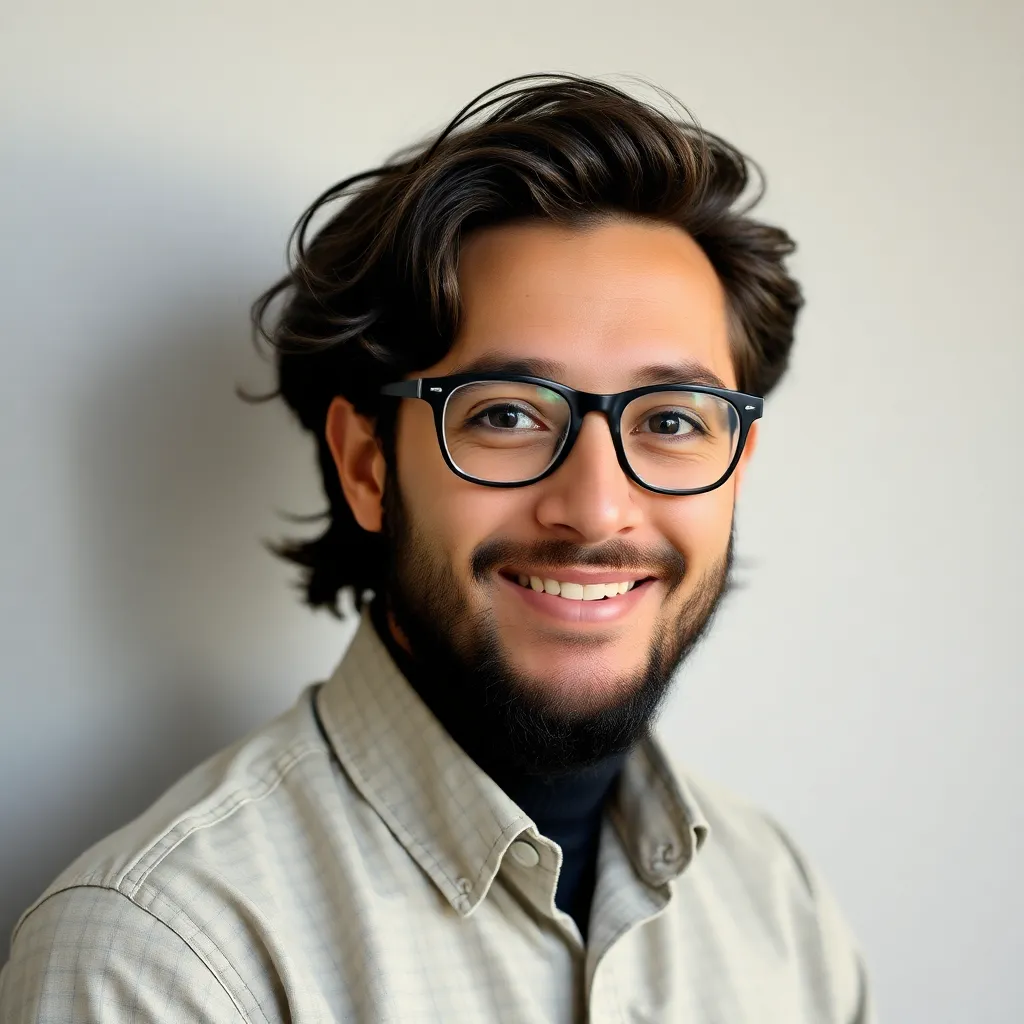
Muz Play
Mar 30, 2025 · 6 min read

Table of Contents
The Flow of Genetic Information in a Cell: From DNA to Protein and Beyond
The central dogma of molecular biology describes the flow of genetic information within a biological system. It posits a unidirectional pathway: DNA makes RNA, and RNA makes protein. While elegantly simple, this foundational principle has evolved with our deeper understanding of molecular mechanisms, revealing complexities and exceptions that enrich our appreciation of cellular processes. This article delves into the intricacies of this flow, exploring the processes of DNA replication, transcription, translation, and the emerging roles of non-coding RNAs, highlighting their significance in cellular function and regulation.
DNA Replication: The Faithful Duplication of the Genetic Blueprint
Before discussing the flow of genetic information, we must first understand how the genetic blueprint itself is duplicated. DNA replication is a fundamental process ensuring the accurate transmission of genetic material during cell division. This semi-conservative process involves unwinding the double helix, separating the two strands, and using each strand as a template to synthesize a new complementary strand.
Key Enzymes and Processes:
- Helicase: Unwinds the DNA double helix at the replication fork, creating a single-stranded template.
- Single-stranded binding proteins (SSBs): Prevent the separated strands from reannealing.
- Primase: Synthesizes short RNA primers, providing a starting point for DNA polymerase.
- DNA polymerase: Adds nucleotides to the 3' end of the growing strand, extending the primer. It possesses proofreading capabilities, minimizing errors during replication.
- Ligase: Joins Okazaki fragments (short DNA sequences synthesized on the lagging strand) to form a continuous strand.
- Topoisomerase: Relieves torsional stress ahead of the replication fork, preventing supercoiling.
The accuracy of DNA replication is crucial for maintaining genome integrity. The inherent proofreading ability of DNA polymerase and other repair mechanisms minimize errors, although mutations can still occur, contributing to genetic diversity and evolution. Understanding the intricacies of DNA replication is foundational to comprehending the subsequent steps in the flow of genetic information.
Transcription: From DNA to RNA
Transcription is the process of synthesizing RNA from a DNA template. This crucial step marks the transition from the stable, long-term storage of genetic information in DNA to the more transient, functional RNA molecules. The enzyme responsible for transcription is RNA polymerase.
Stages of Transcription:
- Initiation: RNA polymerase binds to a specific region of DNA called the promoter, initiating transcription. Promoters contain specific sequences recognized by the polymerase.
- Elongation: RNA polymerase unwinds the DNA double helix and synthesizes a complementary RNA molecule, using one DNA strand as a template. The RNA molecule synthesized is called messenger RNA (mRNA) if it codes for a protein.
- Termination: Transcription ends at a specific termination sequence, releasing the newly synthesized RNA molecule.
The newly synthesized mRNA molecule undergoes several processing steps in eukaryotes, including:
- Capping: Addition of a 5' cap, protecting the mRNA from degradation and aiding in ribosome binding.
- Splicing: Removal of introns (non-coding sequences) and joining of exons (coding sequences). This process generates a mature mRNA molecule ready for translation.
- Polyadenylation: Addition of a poly(A) tail to the 3' end, further protecting the mRNA from degradation and influencing its stability and translation efficiency.
Translation: From RNA to Protein
Translation is the final step in the central dogma, where the genetic code carried by mRNA is translated into a polypeptide chain, forming a protein. This process occurs in the ribosomes, cellular structures composed of ribosomal RNA (rRNA) and proteins. Transfer RNA (tRNA) molecules play a crucial role, acting as adaptors, bringing the appropriate amino acids to the ribosome based on the mRNA codon sequence.
The Ribosome's Role:
The ribosome functions as a molecular machine, facilitating the precise pairing of codons on mRNA with anticodons on tRNA. This pairing determines the amino acid sequence of the growing polypeptide chain. The ribosome consists of two subunits, the small subunit which binds to mRNA and tRNA, and the large subunit which catalyzes peptide bond formation.
Steps in Translation:
- Initiation: The ribosome binds to the mRNA and initiator tRNA, establishing the reading frame.
- Elongation: The ribosome moves along the mRNA, sequentially reading codons and adding amino acids to the growing polypeptide chain. This process involves codon recognition, peptide bond formation, and translocation.
- Termination: Translation ends when a stop codon is encountered, releasing the completed polypeptide chain.
The newly synthesized polypeptide chain then folds into a specific three-dimensional structure, determined by its amino acid sequence and interactions with chaperone proteins, ultimately defining its function.
Beyond the Central Dogma: The Expanding Roles of Non-Coding RNAs
While the central dogma provides a foundational understanding of gene expression, our understanding has significantly evolved. A significant portion of the genome is transcribed into non-coding RNAs (ncRNAs), which play diverse regulatory roles. These ncRNAs don't code for proteins but instead exert their effects through various mechanisms.
Types and Functions of ncRNAs:
-
microRNAs (miRNAs): These short ncRNAs regulate gene expression post-transcriptionally by binding to target mRNAs, leading to their degradation or translational repression. They are involved in various cellular processes, including development, differentiation, and disease.
-
Small interfering RNAs (siRNAs): Similar to miRNAs, siRNAs also regulate gene expression post-transcriptionally, often through RNA interference (RNAi). They are implicated in antiviral defense and gene silencing.
-
Long non-coding RNAs (lncRNAs): These longer ncRNAs exhibit diverse functions, including acting as scaffolds, guides, or decoys to regulate gene expression at multiple levels. They are involved in numerous cellular processes, including chromatin remodeling, transcription regulation, and splicing.
The Dynamic Nature of Genetic Information Flow: Regulation and Feedback
The flow of genetic information is not a static, linear process. It is subject to intricate regulatory mechanisms ensuring that genes are expressed at the appropriate time and in the appropriate amounts. These regulatory mechanisms operate at multiple levels, influencing DNA replication, transcription, and translation.
Regulatory Mechanisms:
-
Transcriptional regulation: This involves controlling the initiation of transcription through the action of transcription factors that bind to specific DNA sequences, either activating or repressing gene expression.
-
Post-transcriptional regulation: This includes processing of mRNA transcripts, RNA stability, and translational control. miRNAs and siRNAs are major players in this stage, influencing the abundance and translational efficiency of target mRNAs.
-
Epigenetic regulation: This involves modifications to DNA or histones that alter chromatin structure and gene expression without changing the DNA sequence itself. These modifications can be heritable and influence gene expression patterns across generations.
The complex interplay of these regulatory mechanisms ensures precise control over gene expression, adapting cellular responses to changing environmental conditions and developmental cues.
Conclusion: A Complex and Dynamic System
The flow of genetic information, from DNA to RNA to protein, forms the basis of life. While the central dogma provides a simplified framework, the reality is much more intricate and dynamic. The discovery and characterization of non-coding RNAs and the understanding of complex regulatory mechanisms have significantly expanded our appreciation of this fundamental biological process. Continued research into the intricacies of gene expression will undoubtedly uncover further complexities and reveal novel regulatory layers that govern cellular function and ultimately, life itself. This deeper understanding is critical not only for fundamental biological research but also for advancing medical and biotechnological applications, addressing diseases caused by defects in gene expression and harnessing the power of gene regulation for therapeutic interventions.
Latest Posts
Latest Posts
-
All Regions Of Earth Where Organisms Live
Apr 01, 2025
-
Active Sites On The Actin Become Available For Binding After
Apr 01, 2025
-
Is Sodium Methoxide A Strong Nucleophile
Apr 01, 2025
-
Which Statement About Immigration Federalism Is False
Apr 01, 2025
-
Which Will Increase The Rate Of A Chemical Reaction
Apr 01, 2025
Related Post
Thank you for visiting our website which covers about The Flow Of Genetic Information In A Cell Goes From . We hope the information provided has been useful to you. Feel free to contact us if you have any questions or need further assistance. See you next time and don't miss to bookmark.