What Controls What Enters And Leaves A Cell
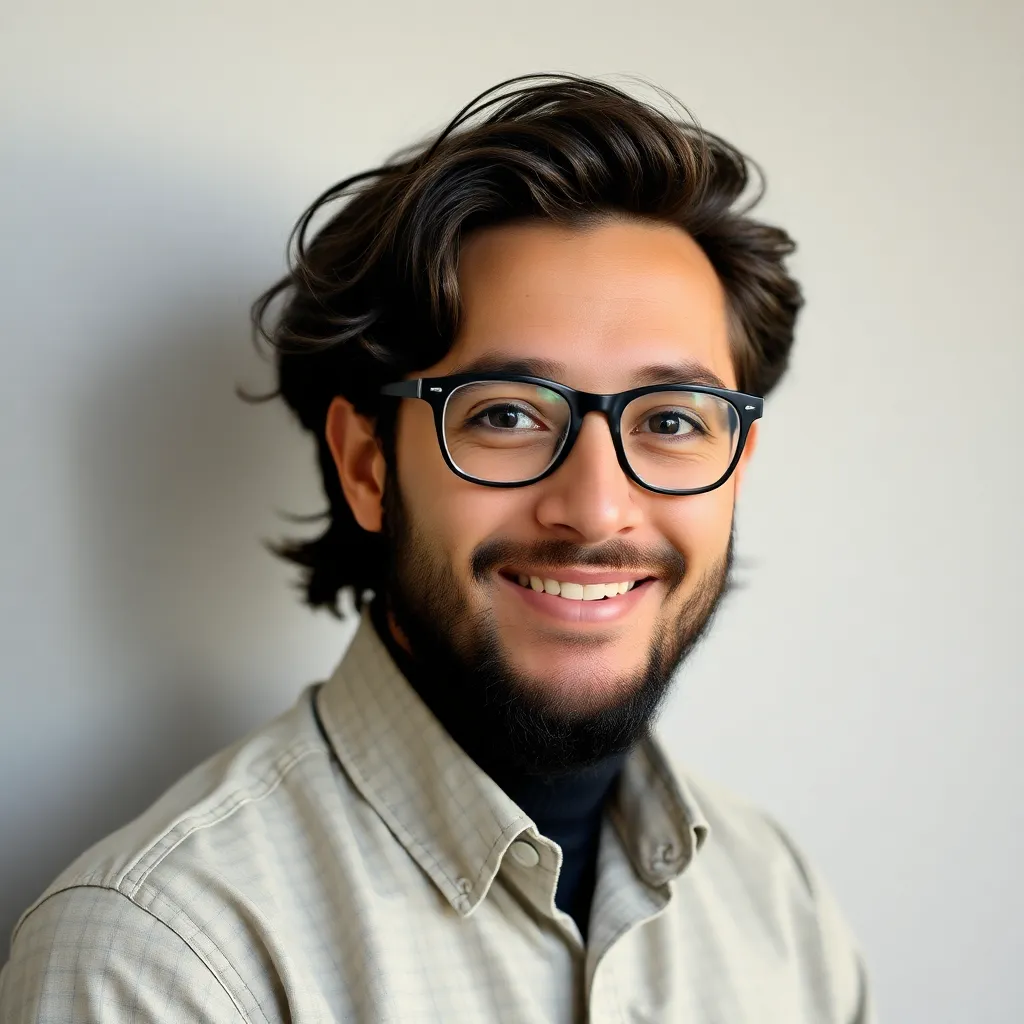
Muz Play
Mar 28, 2025 · 6 min read
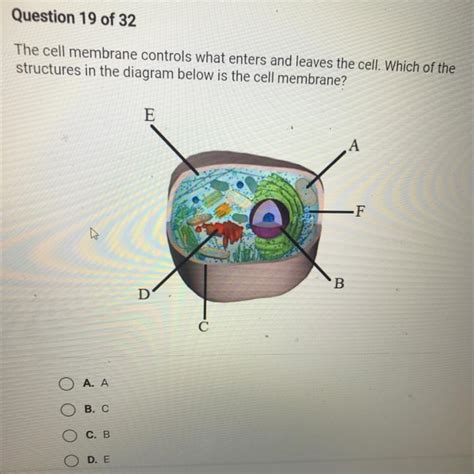
Table of Contents
What Controls What Enters and Leaves a Cell? A Deep Dive into Cell Membranes
The cell, the fundamental unit of life, is a marvel of organization and efficiency. Within its confines, a complex symphony of chemical reactions takes place, constantly building, breaking down, and transforming molecules. But this carefully orchestrated chaos wouldn't be possible without a highly selective gatekeeper: the cell membrane. This remarkable structure controls what enters and leaves the cell, meticulously regulating the internal environment and ensuring the cell's survival. This article delves deep into the mechanisms that govern this crucial process, exploring the structure of the membrane and the various transport methods employed.
The Cell Membrane: A Dynamic Barrier
The cell membrane, also known as the plasma membrane, is not merely a static barrier but a dynamic, fluid mosaic of lipids and proteins. This fluid mosaic model describes the membrane as a two-dimensional liquid where components can move laterally. Its key structural component is the phospholipid bilayer.
Phospholipid Bilayer: The Foundation
Phospholipids are amphipathic molecules, meaning they possess both hydrophilic (water-loving) and hydrophobic (water-fearing) regions. Each phospholipid molecule has a hydrophilic phosphate head and two hydrophobic fatty acid tails. In an aqueous environment, these molecules spontaneously arrange themselves into a bilayer, with the hydrophilic heads facing the watery intracellular and extracellular environments, and the hydrophobic tails tucked away in the interior, shielded from water. This bilayer forms the fundamental structure of the membrane, providing a selectively permeable barrier. The fluidity of this bilayer is crucial, allowing for movement of membrane components and the flexibility required for cell processes like division and signaling. The degree of fluidity is influenced by factors such as temperature and the types of fatty acids present in the phospholipids. Unsaturated fatty acids with kinks in their tails increase fluidity, while saturated fatty acids pack more tightly, decreasing fluidity.
Membrane Proteins: The Gatekeepers and More
Embedded within the phospholipid bilayer are various types of proteins, which are responsible for a wide array of functions, including transport, cell signaling, and enzymatic activity. These proteins are not static; they move laterally within the membrane, contributing to its dynamic nature. These proteins can be classified into two broad categories: integral proteins and peripheral proteins.
-
Integral Proteins: These proteins are embedded within the phospholipid bilayer, often spanning the entire membrane (transmembrane proteins). They have hydrophobic regions that interact with the lipid tails and hydrophilic regions that interact with the aqueous environments. Many integral proteins act as channels or carriers, facilitating the transport of specific molecules across the membrane.
-
Peripheral Proteins: These proteins are associated with the membrane surface, either bound to integral proteins or to the phospholipid heads. They often play roles in cell signaling and structural support.
Cholesterol: Maintaining Membrane Fluidity
Cholesterol, a sterol molecule, is another crucial component of the cell membrane, particularly in animal cells. It is interspersed among the phospholipids, influencing membrane fluidity. At higher temperatures, cholesterol restricts phospholipid movement, decreasing fluidity. Conversely, at lower temperatures, it prevents the phospholipids from packing too tightly, maintaining fluidity and preventing the membrane from solidifying.
Transport Across the Cell Membrane: A Multifaceted Process
The cell membrane's selectivity is achieved through a variety of transport mechanisms, broadly categorized as passive transport and active transport.
Passive Transport: Moving with the Gradient
Passive transport processes do not require energy input from the cell. Movement of molecules occurs down their concentration gradient (from an area of high concentration to an area of low concentration) or down their electrochemical gradient (considering both concentration and electrical charge).
-
Simple Diffusion: Small, nonpolar molecules, such as oxygen (O2) and carbon dioxide (CO2), can simply diffuse across the phospholipid bilayer. Their hydrophobic nature allows them to easily pass through the lipid core.
-
Facilitated Diffusion: Larger or polar molecules, which cannot readily cross the lipid bilayer, require the assistance of membrane proteins. These proteins act as channels or carriers, providing pathways for specific molecules to move across the membrane down their concentration gradient.
-
Channel Proteins: These proteins form hydrophilic pores or channels that allow specific ions or molecules to pass through. Some channels are always open, while others are gated, opening or closing in response to specific stimuli, such as changes in voltage or the binding of a ligand.
-
Carrier Proteins: These proteins bind to specific molecules, undergo a conformational change, and then release the molecule on the other side of the membrane. This process is highly selective, ensuring that only specific molecules are transported.
-
-
Osmosis: This is the passive movement of water across a selectively permeable membrane from a region of high water concentration (low solute concentration) to a region of low water concentration (high solute concentration). Osmosis is crucial for maintaining cell volume and turgor pressure.
Active Transport: Working Against the Gradient
Active transport processes require energy input, typically in the form of ATP, to move molecules against their concentration gradient (from an area of low concentration to an area of high concentration). This movement requires specific transport proteins called pumps.
-
Primary Active Transport: This directly utilizes ATP hydrolysis to move molecules against their gradient. A classic example is the sodium-potassium pump (Na+/K+ pump), which maintains the electrochemical gradient across the cell membrane by pumping three sodium ions (Na+) out of the cell and two potassium ions (K+) into the cell for every ATP molecule hydrolyzed.
-
Secondary Active Transport: This indirectly utilizes energy stored in an electrochemical gradient established by primary active transport. For example, the movement of glucose into intestinal cells is coupled to the movement of sodium ions down their concentration gradient.
Vesicular Transport: Bulk Transport
Vesicular transport involves the movement of large molecules or groups of molecules across the membrane via membrane-bound vesicles. This process requires energy.
-
Endocytosis: This process involves the engulfment of extracellular material by the cell membrane, forming a vesicle that carries the material into the cell. There are three main types of endocytosis: phagocytosis (cell eating), pinocytosis (cell drinking), and receptor-mediated endocytosis (specific uptake of molecules).
-
Exocytosis: This is the reverse of endocytosis, involving the fusion of vesicles containing intracellular material with the cell membrane, releasing the contents to the extracellular environment. Exocytosis is crucial for secretion of hormones, neurotransmitters, and other molecules.
Regulation of Transport: Maintaining Cellular Homeostasis
The precise regulation of transport across the cell membrane is critical for maintaining cellular homeostasis—the stable internal environment necessary for cell survival. This regulation is achieved through various mechanisms, including:
-
Regulation of protein activity: The activity of transport proteins can be modulated by factors such as phosphorylation, ligand binding, or changes in membrane potential.
-
Regulation of protein expression: The number of transport proteins in the membrane can be altered by changes in gene expression.
-
Regulation of membrane fluidity: Changes in membrane fluidity can affect the activity and function of transport proteins.
Conclusion: A Complex and Dynamic System
The control of what enters and leaves a cell is a complex and highly regulated process. The cell membrane, with its fluid mosaic structure and diverse array of transport mechanisms, serves as a dynamic barrier, precisely controlling the passage of molecules to maintain cellular homeostasis. Understanding these mechanisms is crucial to comprehending the fundamental processes of life, as well as developing treatments for diseases that involve malfunctions in cellular transport. Further research continues to reveal the intricate details of this vital cellular function, promising advancements in our understanding of biology and medicine.
Latest Posts
Latest Posts
-
Electric Field Of A Charged Disk
Mar 31, 2025
-
5 Blind Man And The Elephant
Mar 31, 2025
-
Which Statement Describes The Citric Acid Cycle
Mar 31, 2025
-
Why Do Plants Love Water In Bio Terms
Mar 31, 2025
-
Identifying The Important Intermolecular Forces In Pure Compounds
Mar 31, 2025
Related Post
Thank you for visiting our website which covers about What Controls What Enters And Leaves A Cell . We hope the information provided has been useful to you. Feel free to contact us if you have any questions or need further assistance. See you next time and don't miss to bookmark.