What Happens To Pyruvic Acid During The Krebs Cycle
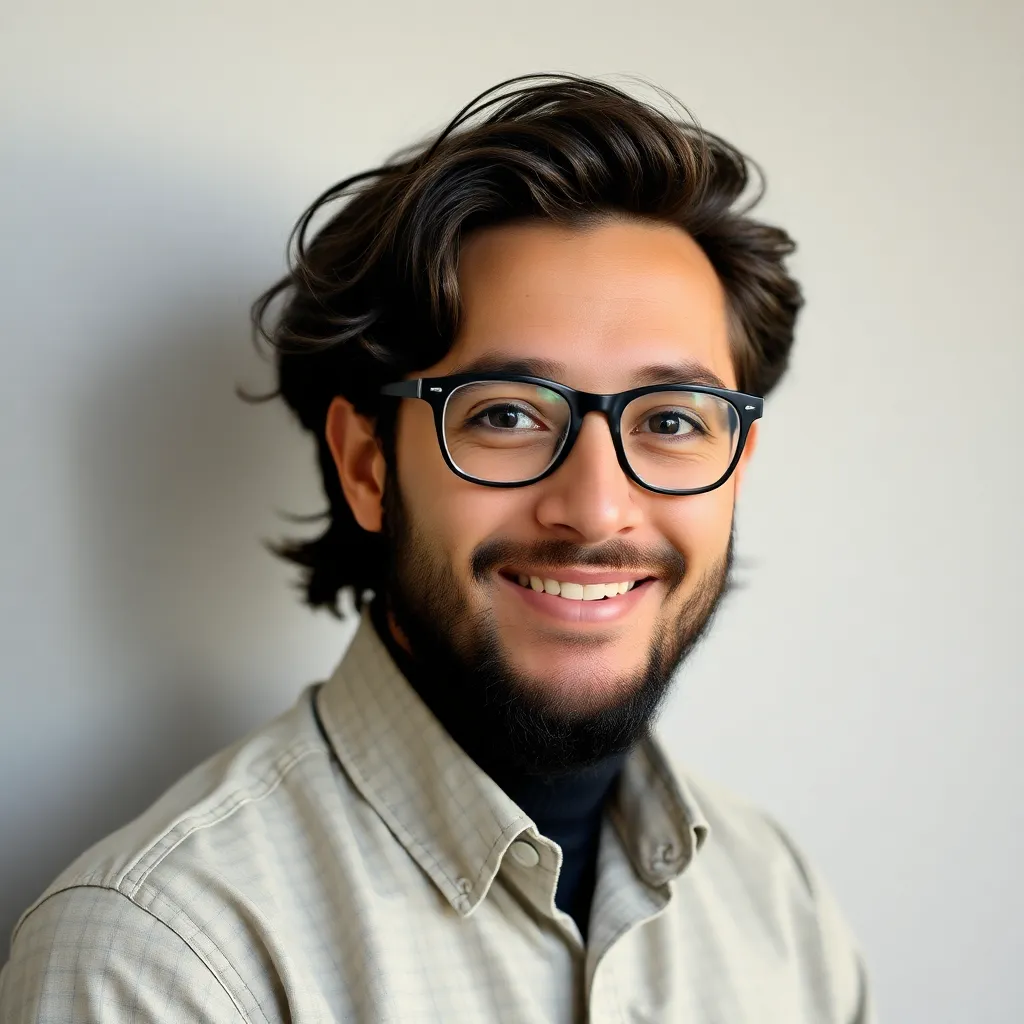
Muz Play
Apr 05, 2025 · 5 min read
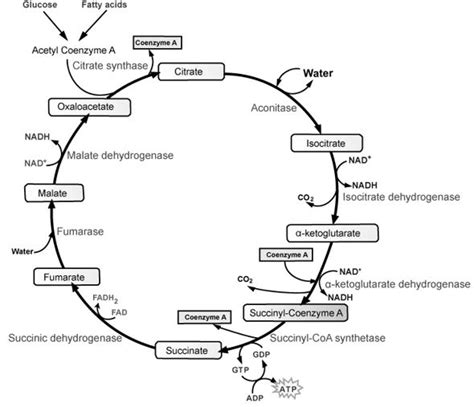
Table of Contents
What Happens to Pyruvic Acid During the Krebs Cycle? A Deep Dive into Cellular Respiration
The Krebs cycle, also known as the citric acid cycle or tricarboxylic acid (TCA) cycle, is a central metabolic pathway found in all aerobic organisms. It's a crucial stage in cellular respiration, the process by which cells break down glucose and other fuel molecules to generate energy in the form of ATP (adenosine triphosphate). But before the Krebs cycle can even begin, pyruvic acid, the end product of glycolysis, needs to undergo a preparatory step. Understanding what happens to pyruvic acid is key to understanding the entire process of cellular respiration.
From Glycolysis to the Krebs Cycle: The Pyruvate Dehydrogenase Complex
Glycolysis, the breakdown of glucose in the cytoplasm, yields two molecules of pyruvic acid per glucose molecule. However, pyruvic acid itself cannot directly enter the Krebs cycle. It first needs to be converted into acetyl-CoA, a crucial step facilitated by a large multi-enzyme complex called the pyruvate dehydrogenase complex (PDC). This process occurs in the mitochondrial matrix, the innermost compartment of the mitochondria, the powerhouse of the cell.
The Decarboxylation and Oxidation of Pyruvate
The pyruvate dehydrogenase complex catalyzes a series of reactions that transform pyruvate into acetyl-CoA. Let's break down the key steps:
-
Decarboxylation: The first step involves the removal of a carboxyl group (-COO⁻) from pyruvate as carbon dioxide (CO₂). This is a crucial step, as it releases the first molecule of carbon dioxide during cellular respiration.
-
Oxidation: Simultaneously, the remaining two-carbon fragment is oxidized. This means it loses electrons. These electrons are accepted by NAD⁺, reducing it to NADH. NADH is a crucial electron carrier that will later contribute to ATP synthesis in the electron transport chain.
-
Acetyl-CoA Formation: The oxidized two-carbon fragment is then attached to coenzyme A (CoA), forming acetyl-CoA. Acetyl-CoA is now ready to enter the Krebs cycle.
In essence, the pyruvate dehydrogenase complex acts as a gateway, converting the three-carbon pyruvate molecule into a two-carbon acetyl-CoA molecule, releasing carbon dioxide and generating NADH in the process. This conversion is an irreversible step, committing the pyruvate to further oxidation in the Krebs cycle.
The Krebs Cycle: A Detailed Look at Acetyl-CoA's Fate
Now that we understand the fate of pyruvic acid before entering the Krebs cycle, let's delve into the cycle itself. Remember, this cycle occurs twice for each glucose molecule because glycolysis produces two pyruvate molecules.
The Krebs cycle is a cyclical series of eight enzyme-catalyzed reactions, each occurring in the mitochondrial matrix. Here's a step-by-step overview:
-
Citrate Synthesis: Acetyl-CoA, the product of pyruvate oxidation, enters the cycle by combining with oxaloacetate, a four-carbon molecule. This reaction, catalyzed by citrate synthase, forms citrate, a six-carbon molecule, and releases CoA.
-
Citrate Isomerization: Citrate is then isomerized to isocitrate. This isomerization involves the rearrangement of the molecule, preparing it for the next steps.
-
Oxidative Decarboxylation 1: Isocitrate undergoes oxidative decarboxylation, catalyzed by isocitrate dehydrogenase. This means it's both oxidized (loses electrons) and decarboxylated (loses a carbon dioxide molecule). This step generates the second molecule of carbon dioxide and NADH.
-
Oxidative Decarboxylation 2: α-Ketoglutarate, the product of the previous step, undergoes another oxidative decarboxylation, catalyzed by α-ketoglutarate dehydrogenase. This step also generates NADH and a molecule of carbon dioxide. Importantly, this step also generates succinyl-CoA, a high-energy thioester bond.
-
Substrate-Level Phosphorylation: Succinyl-CoA, through a series of reactions involving succinyl-CoA synthetase, undergoes substrate-level phosphorylation, directly generating a molecule of GTP (guanosine triphosphate). GTP is readily convertible to ATP, representing a small amount of ATP produced during the Krebs cycle.
-
Oxidation: Succinate, a four-carbon molecule, is oxidized to fumarate, catalyzed by succinate dehydrogenase. This step generates FADH₂, another electron carrier, which is slightly less efficient than NADH in ATP production.
-
Hydration: Fumarate is hydrated to form malate, adding a water molecule to the structure. This reaction is catalyzed by fumarase.
-
Oxidation: Malate is finally oxidized to oxaloacetate, regenerating the four-carbon molecule that started the cycle. This step generates NADH, completing the cycle.
In summary, the Krebs cycle processes the two-carbon acetyl-CoA derived from pyruvate oxidation, producing a substantial amount of energy-rich molecules:
- ATP (or GTP): Two molecules per glucose molecule (one per pyruvate).
- NADH: Six molecules per glucose molecule (three per pyruvate).
- FADH₂: Two molecules per glucose molecule (one per pyruvate).
- CO₂: Four molecules per glucose molecule (two per pyruvate).
The Importance of NADH and FADH₂
The NADH and FADH₂ molecules generated during the Krebs cycle play a pivotal role in the final stage of cellular respiration, the electron transport chain (ETC). These electron carriers deliver their high-energy electrons to the ETC, initiating a series of redox reactions that ultimately generate a large amount of ATP through chemiosmosis.
Regulation of the Krebs Cycle
The Krebs cycle is tightly regulated to ensure the efficient production of ATP and to prevent wasteful production of intermediates. Several factors influence the rate of the cycle, including:
-
Substrate Availability: The availability of acetyl-CoA and oxaloacetate directly influences the rate of citrate synthesis.
-
Enzyme Inhibition: Key enzymes of the Krebs cycle are subject to allosteric regulation. For instance, ATP inhibits citrate synthase, slowing down the cycle when energy levels are high. NADH also inhibits several enzymes.
-
Citrate Accumulation: High levels of citrate can inhibit phosphofructokinase, a key enzyme in glycolysis, preventing further glucose breakdown when the Krebs cycle is already working at capacity.
The Krebs Cycle in Context: A Vital Part of Cellular Respiration
The Krebs cycle is not an isolated pathway. It's intricately connected to other metabolic processes, including glycolysis, fatty acid oxidation (beta-oxidation), and amino acid metabolism. The cycle serves as a central hub for the catabolism of various fuel molecules.
Understanding what happens to pyruvic acid during the Krebs cycle is fundamental to grasping the intricacies of cellular respiration. The conversion of pyruvate to acetyl-CoA, followed by the cyclical process of oxidation, decarboxylation, and energy capture, generates the vital energy currency ATP, fueling all the cellular processes essential for life. The tight regulation of this central metabolic pathway underscores the cell's remarkable ability to efficiently harness energy from fuel molecules.
Latest Posts
Latest Posts
-
Evaluate The Representation Of Ionic Bonding
Apr 06, 2025
-
Change In Allele Frequency Over Time
Apr 06, 2025
-
Label The Features Of The Bone Tissue
Apr 06, 2025
Related Post
Thank you for visiting our website which covers about What Happens To Pyruvic Acid During The Krebs Cycle . We hope the information provided has been useful to you. Feel free to contact us if you have any questions or need further assistance. See you next time and don't miss to bookmark.