What Is Reversible Process In Thermodynamics
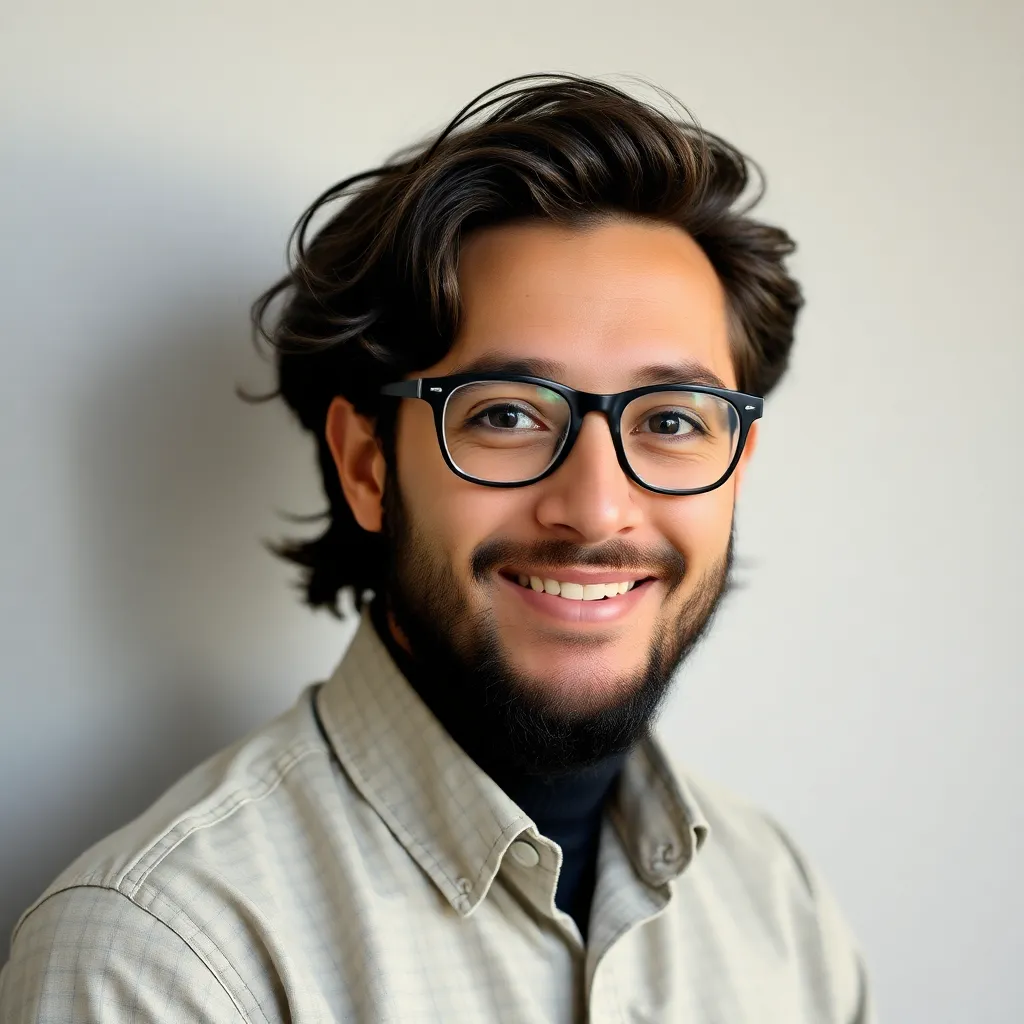
Muz Play
Mar 29, 2025 · 6 min read
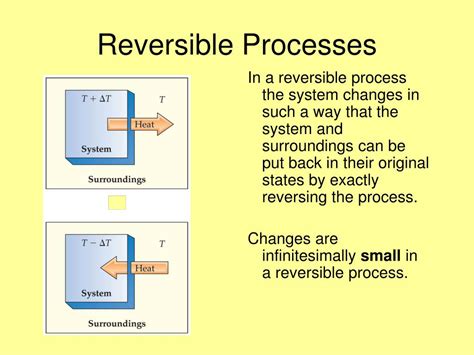
Table of Contents
What is a Reversible Process in Thermodynamics? A Deep Dive
Thermodynamics, the study of heat and its relation to energy and work, introduces many fundamental concepts. Among these, the concept of a reversible process stands out as both crucial and often misunderstood. Understanding reversible processes is essential for grasping the theoretical limits of efficiency in engines and other thermodynamic systems. This comprehensive guide will delve into the intricacies of reversible processes, explaining their characteristics, significance, and limitations.
Defining a Reversible Process
A reversible process, in its simplest definition, is a thermodynamic process that can be reversed without leaving any trace on the surroundings. This means that if the process is reversed, both the system and its surroundings return to their initial states. There is no net change in either the system or the environment after the complete forward and reverse cycle. This idealization is crucial in thermodynamics for establishing theoretical benchmarks against which real-world processes can be compared.
It’s important to note that true reversibility is an idealization. No real-world process is perfectly reversible. Frictional forces, heat losses, and other dissipative effects always accompany real processes, rendering them irreversible to some degree. However, understanding reversible processes is fundamental because they represent the most efficient way a thermodynamic system can operate.
Characteristics of a Reversible Process
Several key characteristics define a reversible process:
1. Infinitesimal Changes
A reversible process occurs through a series of infinitesimal changes in state. This means that at each point along the process, the system is in equilibrium with its surroundings. A change in pressure, temperature, or volume occurs so slowly that the system can adjust instantaneously and remain in equilibrium throughout the entire process. This contrasts sharply with irreversible processes, which occur rapidly and involve significant deviations from equilibrium.
2. Equilibrium Conditions
Throughout a reversible process, the system is always in thermodynamic equilibrium with its surroundings. This implies that there are no significant temperature gradients, pressure differences, or concentration gradients between the system and its surroundings. Any driving force causing the process is infinitesimally small.
3. No Dissipative Effects
Reversible processes are characterized by the absence of dissipative effects. Dissipative effects, such as friction, viscosity, and electrical resistance, generate entropy, which is a measure of disorder in a system. Since reversible processes are entropy-neutral, these dissipative forces must be absent.
4. Path Dependence
While the change in state variables (like internal energy, entropy, enthalpy) between initial and final states is path-independent for a reversible process, the process itself is path-dependent. The exact pathway the system takes through the series of equilibrium states determines the amount of heat and work transferred.
5. Quasi-Static Processes
Reversible processes are often described as quasi-static processes. This term implies that the process proceeds so slowly that the system is always very close to equilibrium. However, it’s important to note that not all quasi-static processes are reversible. A quasi-static process can be irreversible if dissipative forces are present.
Examples of (Theoretically) Reversible Processes
While perfectly reversible processes are hypothetical, some processes closely approximate reversibility under carefully controlled conditions:
-
Isothermal Expansion/Compression of an Ideal Gas: Imagine an ideal gas slowly expanding against a piston in a perfectly insulated container, with the external pressure only infinitesimally less than the internal pressure. The process can be reversed by slowly compressing the gas.
-
Isothermal Expansion of a Gas in a Series of Chambers: A more realistic approximation involves expanding a gas through a series of chambers, each with slightly lower pressure than the previous one. This minimizes dissipative effects.
-
Reversible Phase Transition: A slow and gradual change in temperature or pressure can allow a substance to undergo a phase transition (e.g., melting, boiling) reversibly, with the system remaining in equilibrium at each point.
-
Reversible Chemical Reaction: A chemical reaction conducted under conditions of perfect equilibrium, where the forward and reverse reactions proceed at exactly the same rate, can be considered reversible.
Irreversible Processes: The Contrast
Understanding reversible processes also requires understanding their opposite: irreversible processes. These processes are characterized by:
- Finite changes in state: Irreversible processes proceed rapidly, with significant deviations from equilibrium.
- Presence of dissipative forces: Friction, viscosity, and other dissipative forces are present, leading to entropy generation.
- Path dependence: The amount of work and heat transferred depends not only on the initial and final states but also on the specific path followed.
- Inability to reverse completely: Even if the process is reversed, it leaves a net change in the surroundings. Energy is dissipated as heat, typically increasing the entropy of the universe.
Examples of irreversible processes are numerous and include:
- Free expansion of a gas into a vacuum: The gas expands spontaneously, without doing any work.
- Heat flow between two bodies at different temperatures: Heat spontaneously flows from the hotter to the colder body.
- Mixing of two different gases: The gases mix spontaneously, increasing disorder.
- Inelastic collisions: Kinetic energy is lost as heat during inelastic collisions.
- Most chemical reactions: Chemical reactions are typically irreversible, involving an increase in entropy.
The Importance of Reversible Processes
Despite their idealized nature, reversible processes are vital in thermodynamics for several reasons:
-
Establishing Theoretical Limits: They define the theoretical limits of efficiency for heat engines and other thermodynamic devices. No real-world engine can achieve the same efficiency as a reversible engine operating between the same temperature limits. This is encapsulated in the Carnot Theorem.
-
Calculating Entropy Changes: The change in entropy of a system undergoing a reversible process can be easily calculated using the integral of dQ/T, where dQ is the heat transferred reversibly at temperature T. This provides a benchmark for calculating entropy changes in irreversible processes.
-
Simplifying Calculations: While real-world processes are irreversible, approximating them as reversible simplifies thermodynamic calculations significantly. This approximation is often justified when the irreversibilities are small.
-
Understanding Thermodynamic Equilibrium: The concept of reversibility provides a deep understanding of thermodynamic equilibrium. A system at equilibrium is at a state where no reversible process can take place.
Limitations and Applicability
It's crucial to remember the limitations of the reversible process concept:
- Idealization: They are purely theoretical constructs; no real-world process is perfectly reversible.
- Slow Processes: Reversibility often requires incredibly slow processes, which are impractical in many applications.
- Absence of Friction: The complete absence of friction and other dissipative forces is unrealistic in most physical systems.
Conclusion: A Cornerstone of Thermodynamics
Reversible processes, while idealized, play a pivotal role in thermodynamics. They serve as benchmarks for efficiency, simplify calculations, and provide a deeper understanding of equilibrium and entropy. Although real-world processes are inherently irreversible, understanding reversible processes remains essential for accurately modeling and analyzing thermodynamic systems. By grasping this fundamental concept, one gains a stronger foundation for advanced topics in thermodynamics and its applications in various fields, from engineering to materials science and chemistry. The idealized nature of reversibility highlights the inherent limitations of many practical applications while simultaneously offering a critical framework for analysis and comparison.
Latest Posts
Latest Posts
-
Thesis Statement Examples For Literary Analysis
Mar 31, 2025
-
Why Do Atoms Form Bonds With Other Atoms
Mar 31, 2025
-
What Is The Law Of Conservation Of Charge
Mar 31, 2025
-
How Many Sides Does A Parallelogram Have
Mar 31, 2025
-
Elements That Are Gases At Room Temperature
Mar 31, 2025
Related Post
Thank you for visiting our website which covers about What Is Reversible Process In Thermodynamics . We hope the information provided has been useful to you. Feel free to contact us if you have any questions or need further assistance. See you next time and don't miss to bookmark.