What Process Typically Regulates The Enzymes Involved In Metabolic Reactions
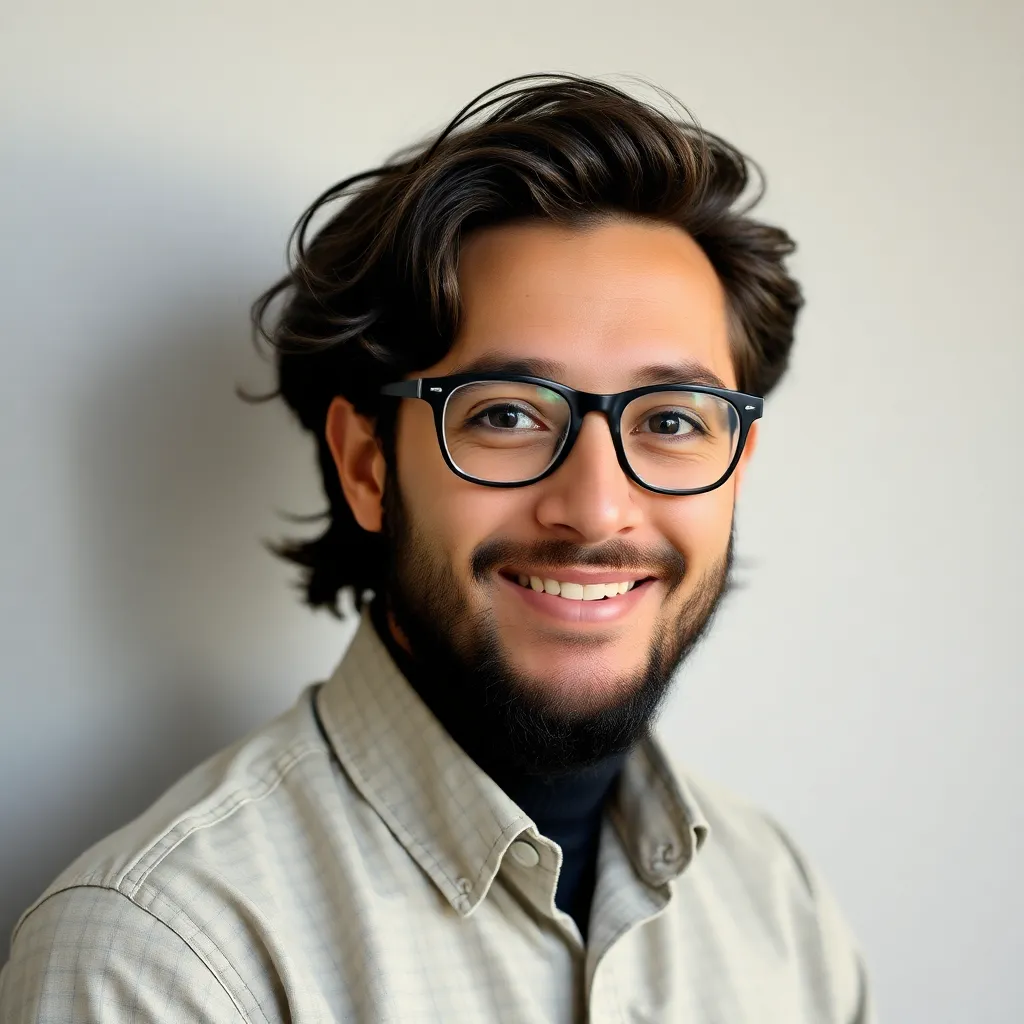
Muz Play
Apr 01, 2025 · 6 min read
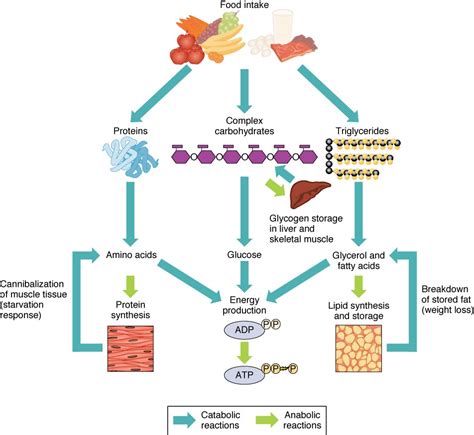
Table of Contents
What Process Typically Regulates the Enzymes Involved in Metabolic Reactions?
Metabolic reactions, the myriad chemical processes sustaining life, are orchestrated by enzymes. These biological catalysts accelerate reaction rates, ensuring efficient energy production, biosynthesis, and waste removal. However, uncontrolled enzyme activity could be detrimental. Therefore, sophisticated regulatory mechanisms are in place to maintain metabolic homeostasis. This article delves into the intricate processes that fine-tune enzyme activity, ensuring a harmonious cellular environment.
Enzyme Regulation: A Multifaceted Approach
Enzyme regulation is crucial for adapting to changing environmental conditions and cellular demands. The cell employs various strategies to control enzyme activity, including:
1. Allosteric Regulation
Allosteric regulation involves the binding of a molecule, called an allosteric effector, to a site on the enzyme distinct from the active site. This binding alters the enzyme's conformation, either enhancing (activation) or inhibiting (inhibition) its catalytic activity.
-
Positive Allosteric Regulation: Binding of an activator stabilizes the enzyme's active conformation, increasing its affinity for the substrate. This is often observed in feedback mechanisms where the end product of a metabolic pathway activates an earlier enzyme.
-
Negative Allosteric Regulation: Binding of an inhibitor stabilizes the enzyme's inactive conformation, reducing its affinity for the substrate. This is a common feedback inhibition mechanism where the end product inhibits an early enzyme in its own pathway, preventing overproduction. Think of it as a self-regulating system to prevent wasteful excess.
Example: Phosphofructokinase (PFK), a key enzyme in glycolysis, is allosterically inhibited by ATP (high energy signal) and activated by AMP (low energy signal). This exquisite sensitivity ensures glycolysis proceeds only when energy is needed.
2. Covalent Modification
Covalent modification involves the attachment or removal of chemical groups to the enzyme, altering its activity. This is a reversible process and a very common method of regulation.
-
Phosphorylation: The most prevalent covalent modification, involving the addition of a phosphate group (PO43-) to serine, threonine, or tyrosine residues. Phosphorylation can either activate or inhibit an enzyme, depending on the specific enzyme and the location of the phosphorylation site. Protein kinases catalyze phosphorylation, while protein phosphatases remove the phosphate group.
-
Acetylation: The addition of an acetyl group (CH3CO-) to lysine residues, often affecting gene expression and metabolic enzymes.
-
Methylation: The addition of a methyl group (CH3-) to various amino acid residues, influencing enzyme activity and protein-protein interactions.
Example: Glycogen phosphorylase, an enzyme that breaks down glycogen, is activated by phosphorylation. This ensures a rapid supply of glucose when energy demands increase.
3. Proteolytic Cleavage
Some enzymes are synthesized as inactive precursors called zymogens or proenzymes. These inactive forms are activated by proteolytic cleavage, where a specific portion of the polypeptide chain is removed, revealing the active site. This is an irreversible activation method, ensuring precise control over the enzyme’s activity, preventing premature activity and potential damage.
Example: Digestive enzymes like trypsinogen and chymotrypsinogen are secreted as inactive zymogens in the pancreas. They are activated in the small intestine by proteolytic cleavage, preventing self-digestion of the pancreas. This is a safety mechanism critical for preventing organ damage.
4. Compartmentalization
Subcellular localization plays a vital role in enzyme regulation. Segregating enzymes within specific cellular compartments ensures their activity is restricted to the appropriate location and prevents unwanted cross-talk between metabolic pathways. This spatial organization promotes efficiency and prevents potentially harmful interactions.
Example: Fatty acid synthesis enzymes are located in the cytoplasm, while fatty acid oxidation enzymes are found in the mitochondria. This separation prevents futile cycling – simultaneous synthesis and breakdown – thus optimizing energy use. The compartmentalization ensures pathways operate in a coordinated manner.
5. Feedback Inhibition
Feedback inhibition is a crucial regulatory mechanism where the end product of a metabolic pathway inhibits an enzyme early in the same pathway. This prevents overproduction of the end product and conserves resources. It's a negative feedback loop, maintaining homeostasis. The product binds to the enzyme allosterically, slowing down the rate.
Example: Threonine dehydratase, an enzyme in threonine metabolism, is inhibited by isoleucine, the end product of the pathway. When sufficient isoleucine is present, the pathway is slowed down, preventing excessive isoleucine production. This efficient mechanism prevents the wasteful creation of excess product.
6. Gene Regulation
The most long-term method of enzyme regulation involves controlling the expression of the genes encoding the enzymes. This affects the overall amount of the enzyme present in the cell. Mechanisms include:
-
Transcriptional Regulation: Controlling the rate of transcription (DNA to RNA) through the action of transcription factors, which bind to promoter regions of the enzyme's gene.
-
Translational Regulation: Controlling the rate of translation (RNA to protein) through factors influencing ribosome binding to mRNA.
Example: During starvation, the expression of genes encoding enzymes involved in gluconeogenesis (glucose synthesis) is increased, ensuring sufficient glucose for the brain and other tissues. Conversely, enzymes involved in glucose breakdown are downregulated. This reflects an elegant long-term adaptation to changing nutritional conditions.
Integration of Regulatory Mechanisms
Enzyme regulation is not a single event but rather a complex interplay of various mechanisms. The cell utilizes a combination of these strategies to achieve precise control over metabolic fluxes. For example, an enzyme might be subject to allosteric regulation, covalent modification, and compartmentalization simultaneously. This layered approach allows for fine-tuning of enzyme activity in response to various stimuli and changing conditions.
Example: The enzyme pyruvate dehydrogenase, a key enzyme in the link between glycolysis and the citric acid cycle, is regulated by phosphorylation (inactivation) and dephosphorylation (activation), allosteric regulation by its substrates and products, and substrate availability. This multi-faceted control ensures that pyruvate is efficiently utilized based on the cell's energetic needs.
Importance of Enzyme Regulation in Disease
Dysregulation of enzyme activity is implicated in numerous diseases. Genetic defects leading to enzyme deficiencies can result in metabolic disorders, such as phenylketonuria (PKU) and galactosemia. Conversely, excessive enzyme activity can also be detrimental, as seen in some cancers where uncontrolled enzyme activity drives uncontrolled cell growth.
Understanding the intricate mechanisms regulating enzymes is crucial for developing therapies targeting metabolic disorders and cancers. This could involve designing drugs that modulate enzyme activity, either enhancing or inhibiting it as needed. The specificity and delicate balance of these regulatory mechanisms highlight the complexity of cellular life and the sophistication of biological control.
Conclusion
The regulation of enzymes involved in metabolic reactions is a complex and crucial process for maintaining cellular homeostasis and responding to environmental changes. A multifaceted approach, utilizing allosteric regulation, covalent modification, proteolytic cleavage, compartmentalization, feedback inhibition, and gene regulation, ensures precise control over enzyme activity. Understanding these regulatory mechanisms is pivotal for comprehending fundamental cellular processes and developing treatments for various diseases associated with enzyme dysregulation. The intricate interplay of these mechanisms ensures metabolic efficiency and adaptability, highlighting the remarkable sophistication of biological systems. Future research will undoubtedly reveal further complexities and nuances in this essential area of cellular biology.
Latest Posts
Latest Posts
-
In Which Phase Of Cellular Respiration Is Water Made
Apr 02, 2025
-
How To Measure Current On A Breadboard
Apr 02, 2025
-
Chapter 1 Lab Investigation The Language Of Anatomy
Apr 02, 2025
-
How Is Chemistry Used In Forensic Science
Apr 02, 2025
-
How Do You Square A Radical
Apr 02, 2025
Related Post
Thank you for visiting our website which covers about What Process Typically Regulates The Enzymes Involved In Metabolic Reactions . We hope the information provided has been useful to you. Feel free to contact us if you have any questions or need further assistance. See you next time and don't miss to bookmark.