Which Electron Carrier S Function In The Citric Acid Cycle
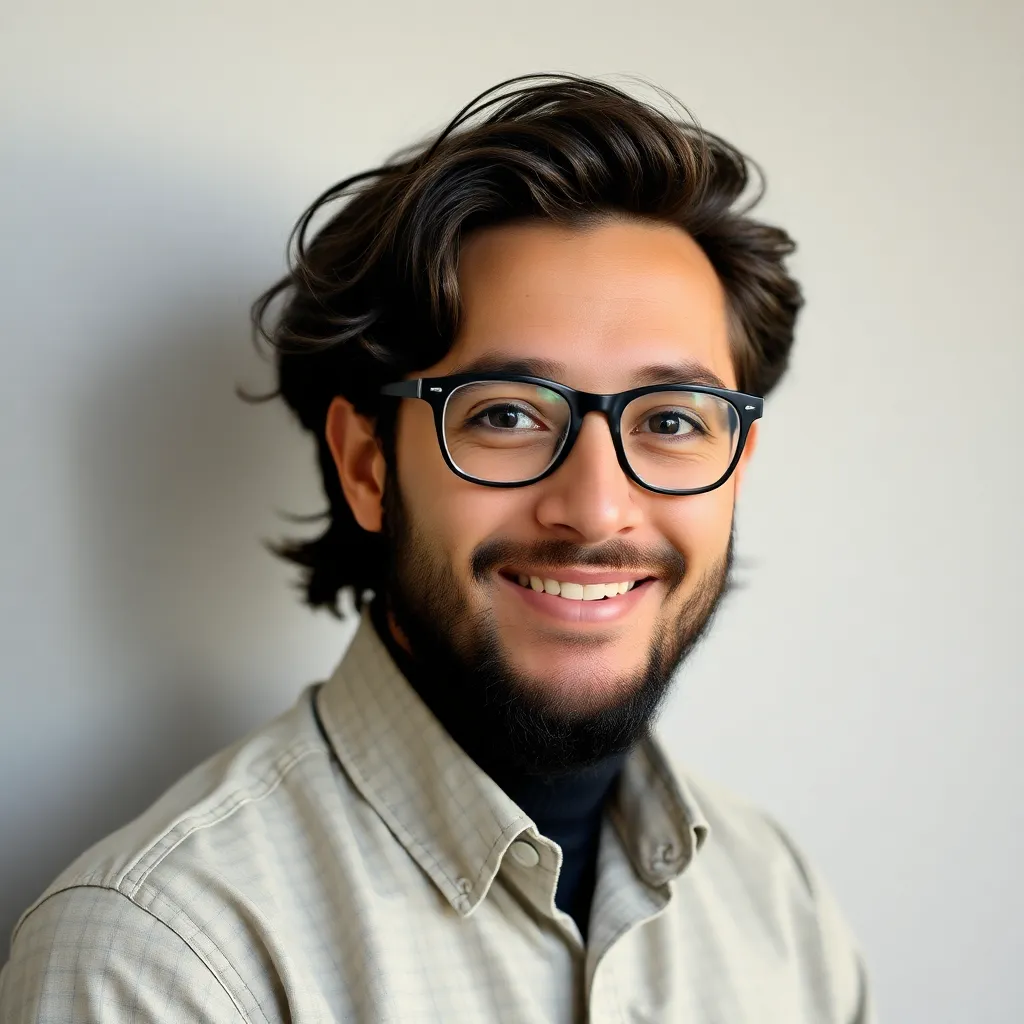
Muz Play
Apr 05, 2025 · 6 min read
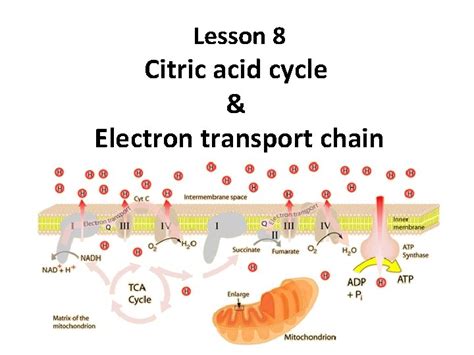
Table of Contents
Which Electron Carriers Function in the Citric Acid Cycle?
The citric acid cycle, also known as the Krebs cycle or tricarboxylic acid (TCA) cycle, is a central metabolic pathway in all aerobic organisms. This crucial cycle plays a vital role in cellular respiration, generating high-energy electron carriers that fuel the electron transport chain, ultimately leading to ATP synthesis – the cell's primary energy currency. Understanding the electron carriers involved is key to grasping the cycle's efficiency and importance. This article will delve deep into the specifics of the electron carriers functioning within the citric acid cycle, examining their roles and significance in energy production.
The Citric Acid Cycle: A Quick Overview
Before focusing on the electron carriers, let's briefly revisit the citric acid cycle itself. This cyclical pathway occurs in the mitochondria of eukaryotic cells and the cytoplasm of prokaryotes. It's a series of eight enzyme-catalyzed reactions that oxidize acetyl-CoA, a two-carbon molecule derived from pyruvate (the end product of glycolysis), to carbon dioxide. This oxidation process is not a simple burning; rather, it's a carefully controlled release of energy, captured in the form of high-energy electron carriers.
The cycle begins with the condensation of acetyl-CoA with oxaloacetate (a four-carbon molecule) to form citrate (a six-carbon molecule). Through a series of isomerizations, dehydrations, and oxidations, citrate is gradually broken down, releasing two molecules of CO₂. Crucially, at specific steps, electrons are extracted and transferred to electron carriers, which then transport these high-energy electrons to the electron transport chain.
Key Electron Carriers in the Citric Acid Cycle
The citric acid cycle utilizes two primary electron carriers:
-
Nicotinamide adenine dinucleotide (NAD⁺): This is arguably the most important electron carrier in the citric acid cycle, as it's reduced to NADH in three separate steps. NAD⁺ accepts two electrons and a proton (H⁺), becoming NADH. This reduced form carries the high-energy electrons to the electron transport chain, where they contribute significantly to ATP production.
-
Flavin adenine dinucleotide (FAD): FAD is another crucial electron carrier, reduced to FADH₂ in one step of the citric acid cycle. Similar to NAD⁺, FAD accepts two electrons and two protons, becoming FADH₂. FADH₂ also delivers its high-energy electrons to the electron transport chain, though at a slightly different point compared to NADH, resulting in a slightly lower ATP yield per molecule.
Detailed Analysis of Electron Carrier Involvement in Each Step
Let's examine each step of the citric acid cycle where electron carriers are involved:
Step 3: Isocitrate Dehydrogenase
This step involves the oxidative decarboxylation of isocitrate to α-ketoglutarate. A molecule of CO₂ is released, and NAD⁺ is reduced to NADH. This is a significant step in the cycle, as it generates the first NADH molecule, carrying high-energy electrons that will eventually contribute to ATP synthesis.
Enzyme: Isocitrate dehydrogenase
Reaction: Isocitrate + NAD⁺ → α-ketoglutarate + NADH + CO₂ + H⁺
Step 4: α-Ketoglutarate Dehydrogenase
This is another oxidative decarboxylation step, converting α-ketoglutarate to succinyl-CoA. Once again, a molecule of CO₂ is released, and NAD⁺ is reduced to NADH. This step is highly regulated and essential for controlling the overall flux through the citric acid cycle.
Enzyme: α-Ketoglutarate dehydrogenase complex
Reaction: α-Ketoglutarate + NAD⁺ + CoA-SH → Succinyl-CoA + NADH + CO₂ + H⁺
Step 6: Succinate Dehydrogenase
This step is unique because it's the only step where FAD is reduced to FADH₂. Succinate is oxidized to fumarate, and FAD accepts the electrons directly, becoming FADH₂. The enzyme, succinate dehydrogenase, is directly embedded in the inner mitochondrial membrane, allowing FADH₂ to directly transfer its electrons to the electron transport chain without the need for further transport. This membrane localization is critical for efficient energy transfer.
Enzyme: Succinate dehydrogenase
Reaction: Succinate + FAD → Fumarate + FADH₂
Step 8: Malate Dehydrogenase
The final step involving an electron carrier in the citric acid cycle is the oxidation of malate to oxaloacetate. Here, NAD⁺ is reduced to NADH, completing the cycle and regenerating oxaloacetate, ready to accept another acetyl-CoA molecule and continue the cycle.
Enzyme: Malate dehydrogenase
Reaction: Malate + NAD⁺ → Oxaloacetate + NADH + H⁺
The Significance of NADH and FADH₂ in ATP Production
The NADH and FADH₂ molecules generated during the citric acid cycle are pivotal for ATP synthesis. They transport their high-energy electrons to the electron transport chain (ETC), located in the inner mitochondrial membrane. The ETC is a series of protein complexes that transfer electrons through a series of redox reactions, ultimately transferring them to oxygen (the final electron acceptor), forming water.
The movement of electrons through the ETC pumps protons (H⁺) across the inner mitochondrial membrane, creating a proton gradient. This proton gradient drives ATP synthesis via chemiosmosis, a process where the protons flow back across the membrane through ATP synthase, an enzyme that uses the energy of this flow to phosphorylate ADP to ATP.
The number of ATP molecules generated per NADH and FADH₂ varies slightly depending on the shuttle system used to transport NADH from the cytosol to the mitochondria and the efficiency of the ETC. However, it's generally accepted that each NADH generates approximately 2.5 ATP molecules, and each FADH₂ generates approximately 1.5 ATP molecules.
Regulation of the Citric Acid Cycle and Electron Carrier Production
The citric acid cycle is meticulously regulated to meet the cell's energy demands. Several factors influence its activity, including:
-
Substrate availability: The concentrations of acetyl-CoA, NAD⁺, and FAD influence the rate of the cycle. High levels of ATP tend to inhibit the cycle, while low levels stimulate it.
-
Product inhibition: High levels of NADH and ATP inhibit several enzymes of the cycle, preventing excessive electron carrier production when energy levels are high.
-
Allosteric regulation: Certain enzymes of the cycle are regulated by allosteric effectors, molecules that bind to the enzyme and alter its activity. For instance, citrate inhibits phosphofructokinase, an enzyme in glycolysis, while ADP and AMP activate it, reflecting the cell's energy status.
The regulation ensures that the production of NADH and FADH₂ is tightly coupled to the cell's energy needs, preventing wasteful overproduction of these electron carriers.
Clinical Significance of Citric Acid Cycle Dysfunction
Disruptions in the citric acid cycle, often resulting from genetic defects or environmental factors, can lead to various metabolic disorders. These disorders frequently manifest as energy deficits and the accumulation of intermediary metabolites. For example, defects in enzyme function within the cycle can lead to the buildup of specific metabolites, causing various clinical symptoms depending on the specific enzyme and metabolite affected.
Conclusion
The citric acid cycle's efficiency hinges on the precise functioning of its electron carriers, NAD⁺ and FAD. These molecules play critical roles in capturing the energy released during the oxidation of acetyl-CoA, transferring high-energy electrons to the electron transport chain, and ultimately driving ATP synthesis. Understanding the detailed involvement of NADH and FADH₂ in each step of the cycle is essential for comprehending cellular respiration and the intricate balance of energy production within living organisms. The tight regulation of this cycle, and thus the production of these crucial electron carriers, underscores its importance in maintaining cellular homeostasis and overall health. Dysfunction in this cycle has significant clinical consequences, highlighting the essential role these electron carriers play in maintaining life's processes.
Latest Posts
Latest Posts
-
Associative Commutative And Distributive Property Examples
Apr 06, 2025
-
Partial Pressure Of Co2 In Air
Apr 06, 2025
-
Example Of Dependent Samples T Test
Apr 06, 2025
-
How To Calculate Interest On A Note Payable
Apr 06, 2025
-
Name The Nitrogenous Bases That Are Classified As Pyrimidines
Apr 06, 2025
Related Post
Thank you for visiting our website which covers about Which Electron Carrier S Function In The Citric Acid Cycle . We hope the information provided has been useful to you. Feel free to contact us if you have any questions or need further assistance. See you next time and don't miss to bookmark.