Which Homeostatic Process Moves Particles Against A Concentration Gradient
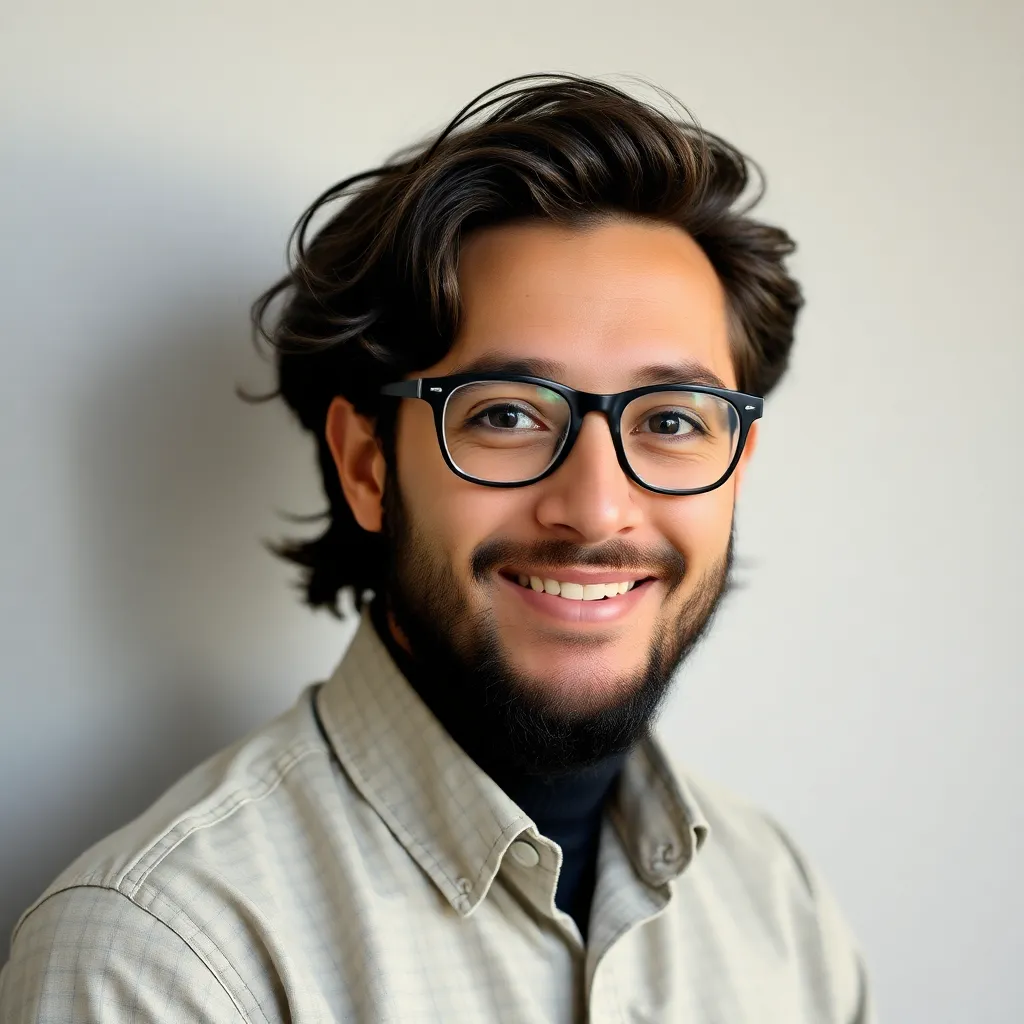
Muz Play
Apr 01, 2025 · 6 min read
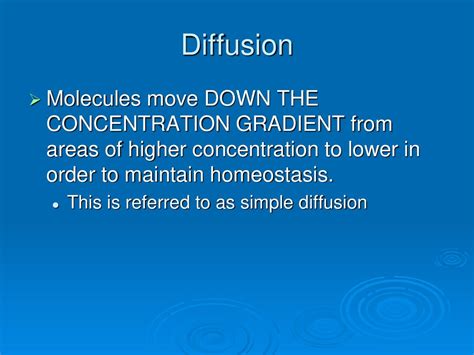
Table of Contents
Which Homeostatic Process Moves Particles Against a Concentration Gradient?
Maintaining a stable internal environment, or homeostasis, is crucial for all living organisms. This involves regulating numerous factors, including the concentration of various substances within cells and the body. One key process that ensures this stability is the movement of particles against a concentration gradient – a process requiring energy expenditure. This article will delve deep into this fascinating and vital aspect of homeostasis, exploring the underlying mechanisms, significance, and various examples in biological systems.
Understanding Concentration Gradients
Before exploring the processes that move particles against concentration gradients, it's essential to understand what a concentration gradient is. A concentration gradient refers to the difference in the concentration of a substance between two areas. Substances naturally tend to move from an area of high concentration to an area of low concentration, a process known as passive transport. This movement continues until equilibrium is reached, where the concentration is uniform throughout. Think of dropping a sugar cube into a glass of water; the sugar will gradually diffuse until it's evenly distributed.
However, many biological processes require the movement of substances against this concentration gradient – from an area of low concentration to an area of high concentration. This is known as active transport, and it's a fundamental aspect of homeostasis that necessitates energy input.
Active Transport: The Key Player in Moving Particles Against a Concentration Gradient
Active transport is the homeostatic process that facilitates the movement of particles against their concentration gradient. This process is energy-dependent, primarily utilizing adenosine triphosphate (ATP) as the energy source. ATP is the primary energy currency of cells, and its hydrolysis (breaking down into ADP and inorganic phosphate) releases the energy needed to power active transport mechanisms.
Several key characteristics define active transport:
- Energy Requirement: Unlike passive transport, active transport requires energy to function. This energy is usually derived from ATP hydrolysis.
- Specificity: Active transport systems are highly specific. They typically only transport certain types of molecules or ions. This specificity is often mediated by transport proteins, embedded within cell membranes.
- Saturation: Active transport systems can become saturated. This means that there's a limit to the rate at which they can transport substances. Once all the transport proteins are occupied, increasing the concentration of the transported substance will not increase the transport rate further.
- Against the Gradient: The defining feature of active transport is that it moves substances against their concentration gradient.
Mechanisms of Active Transport
Several mechanisms facilitate active transport, each with unique characteristics:
1. Primary Active Transport
Primary active transport directly utilizes the energy released from ATP hydrolysis to move particles against their concentration gradient. A prime example is the sodium-potassium pump (Na+/K+ ATPase), found in the cell membranes of virtually all animal cells. This pump maintains the crucial electrochemical gradient across the cell membrane, crucial for nerve impulse transmission, muscle contraction, and many other cellular processes. The pump moves three sodium ions (Na+) out of the cell and two potassium ions (K+) into the cell for every ATP molecule hydrolyzed.
2. Secondary Active Transport
Secondary active transport uses the energy stored in an electrochemical gradient established by primary active transport to move other substances against their concentration gradient. This doesn't directly involve ATP hydrolysis; instead, it leverages the potential energy stored in the gradient created by primary active transport. There are two main types of secondary active transport:
-
Symport: In symport, two substances move across the membrane in the same direction. One substance moves down its concentration gradient (providing the energy), and the other moves against its concentration gradient. For example, the absorption of glucose in the intestines relies on a symporter that couples glucose transport with sodium ion transport.
-
Antiport: In antiport, two substances move across the membrane in opposite directions. One moves down its concentration gradient, and the other moves against its gradient. The sodium-calcium exchanger is a classic example of antiport, where calcium ions are pumped out of the cell against their concentration gradient while sodium ions move into the cell down their concentration gradient.
Importance of Active Transport in Homeostasis
Active transport is vital for maintaining homeostasis in numerous ways:
-
Maintaining Cell Volume: Active transport regulates the movement of ions across cell membranes, preventing osmotic imbalances that could lead to cell swelling or shrinkage.
-
Nutrient Uptake: Active transport is essential for absorbing nutrients from the environment. For instance, plants use active transport to absorb minerals from the soil, while animals absorb nutrients from the digestive tract.
-
Waste Removal: Active transport plays a crucial role in excreting metabolic waste products from cells.
-
Neurotransmission: The sodium-potassium pump is crucial for generating the nerve impulses that underlie all nervous system function.
-
Muscle Contraction: Active transport of calcium ions is vital for muscle contraction and relaxation.
-
Maintaining pH Balance: Active transport of protons (H+) contributes to maintaining the pH balance within cells and the body.
Examples of Active Transport in Different Biological Systems
Active transport is a ubiquitous process, playing crucial roles in various biological systems:
1. Intestinal Absorption: The absorption of glucose, amino acids, and other essential nutrients from the digestive tract relies heavily on active transport mechanisms, particularly secondary active transport coupled with sodium ion gradients.
2. Renal Tubule Function: The kidneys utilize active transport extensively to reabsorb essential substances from the filtrate and excrete waste products. The reabsorption of glucose and amino acids in the proximal convoluted tubule is a prime example.
3. Nerve Impulse Transmission: The sodium-potassium pump is the cornerstone of nerve impulse transmission. Its activity maintains the resting membrane potential, enabling the generation and propagation of action potentials.
4. Plant Root Systems: Plants use active transport to absorb essential minerals from the soil against their concentration gradients. This process is crucial for their growth and survival.
5. Bacterial Transport Systems: Bacteria also utilize active transport to take up nutrients and expel waste products, showcasing the universality of this process across different domains of life.
Dysfunction of Active Transport and Associated Diseases
The proper functioning of active transport is essential for health. Dysfunction in these mechanisms can lead to various diseases:
-
Cystic Fibrosis: This genetic disorder affects the function of chloride ion channels, leading to the accumulation of thick mucus in the lungs and other organs.
-
Familial Hypercholesterolemia: Mutations in genes encoding LDL receptors, responsible for active transport of cholesterol into cells, lead to high cholesterol levels in the blood, increasing the risk of cardiovascular diseases.
-
Cardiac Arrhythmias: Disruptions in ion transport, especially involving sodium and potassium channels, can lead to irregular heartbeats.
Conclusion
Active transport, the homeostatic process that moves particles against a concentration gradient, is a fundamental and ubiquitous process essential for life. This energy-dependent process plays a vital role in maintaining cellular and organismal homeostasis by regulating the concentration of various substances, enabling nutrient uptake, waste removal, and countless other essential biological functions. Understanding the mechanisms and significance of active transport is crucial to comprehending the complexity and elegance of biological systems and the impact of their dysfunction on human health. Future research in this field continues to unravel the intricacies of active transport and its broader implications for various physiological processes and diseases.
Latest Posts
Latest Posts
-
How To Measure Current On A Breadboard
Apr 02, 2025
-
Chapter 1 Lab Investigation The Language Of Anatomy
Apr 02, 2025
-
How Is Chemistry Used In Forensic Science
Apr 02, 2025
-
How Do You Square A Radical
Apr 02, 2025
-
Was Adolf Hitler A Good Leader
Apr 02, 2025
Related Post
Thank you for visiting our website which covers about Which Homeostatic Process Moves Particles Against A Concentration Gradient . We hope the information provided has been useful to you. Feel free to contact us if you have any questions or need further assistance. See you next time and don't miss to bookmark.