5 Conditions Of Hardy Weinberg Equilibrium
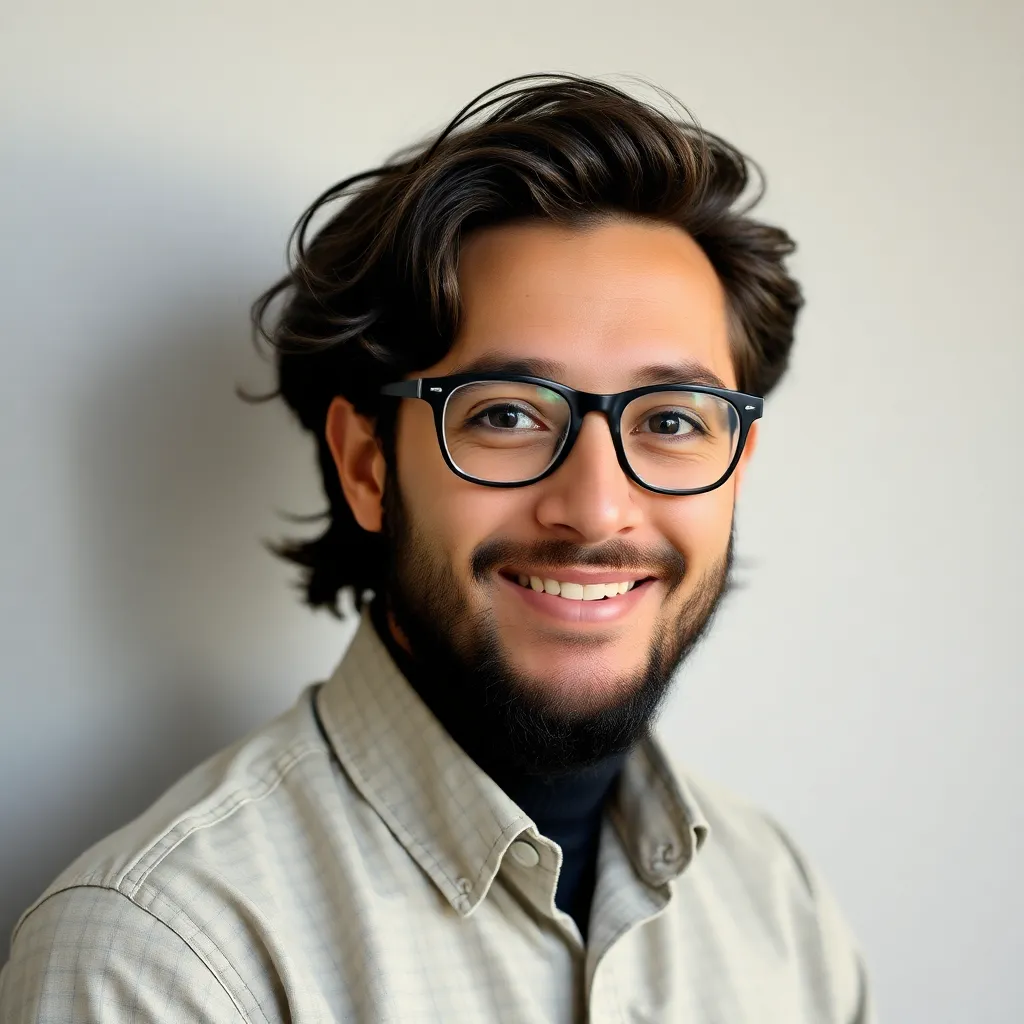
Muz Play
Apr 05, 2025 · 6 min read
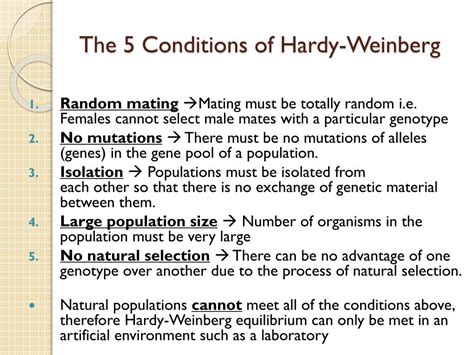
Table of Contents
5 Conditions of Hardy-Weinberg Equilibrium: A Deep Dive
The Hardy-Weinberg principle, also known as the Hardy-Weinberg equilibrium, is a fundamental concept in population genetics. It describes a theoretical population that is not evolving, meaning the allele and genotype frequencies remain constant from generation to generation. Understanding this principle provides a baseline against which to measure real-world population changes and identify evolutionary forces at play. While rarely perfectly observed in nature, it serves as a crucial model for understanding the mechanisms of evolution. This principle rests on five key conditions. If any of these conditions are violated, the population will deviate from Hardy-Weinberg equilibrium, indicating that evolutionary processes are affecting the gene pool.
The Five Pillars of Hardy-Weinberg Equilibrium
The Hardy-Weinberg equilibrium rests upon five fundamental conditions:
- No Mutation: The rate of mutation must be negligible. Mutations introduce new alleles into the population, altering allele frequencies.
- Random Mating: Individuals must mate randomly, without any preference for certain genotypes. Non-random mating, such as assortative mating (mating with similar individuals) or disassortative mating (mating with dissimilar individuals), can significantly alter genotype frequencies.
- No Gene Flow: There should be no migration of individuals into or out of the population. Gene flow introduces or removes alleles, changing the allele frequencies within the population.
- No Genetic Drift: The population must be large enough to avoid the effects of genetic drift. Genetic drift, the random fluctuation of allele frequencies due to chance events, is more pronounced in small populations and can lead to significant changes in allele frequencies over time.
- No Natural Selection: All genotypes must have equal survival and reproductive rates. Natural selection favors certain genotypes over others, leading to changes in allele frequencies as advantageous alleles become more common.
Let's delve deeper into each of these conditions:
1. No Mutation: The Stability of the Gene Pool
Mutations are spontaneous changes in the DNA sequence. They represent the ultimate source of genetic variation within a population. While the rate of mutation for any given gene is typically low, the cumulative effect of mutations across many genes and over many generations can be substantial. If mutations occur frequently, they introduce new alleles into the population, shifting allele frequencies away from the equilibrium state. This disruption to the Hardy-Weinberg equilibrium is particularly significant if the mutation provides a selective advantage or disadvantage. A beneficial mutation, for example, will increase in frequency over time through natural selection, a clear departure from the equilibrium expectation.
Consequences of Mutation: Mutations can lead to the introduction of completely new alleles, altering the existing genetic diversity. This can result in new phenotypes and potential adaptations. However, in the context of the Hardy-Weinberg equilibrium, the key is the rate of mutation. A low mutation rate will have minimal impact on equilibrium, while a high rate will significantly disrupt it.
2. Random Mating: The Absence of Mate Choice
Random mating implies that every individual in the population has an equal chance of mating with any other individual, regardless of their genotype. This random pairing ensures that allele combinations are determined solely by chance and not influenced by mate selection. However, in reality, many organisms exhibit non-random mating patterns.
Types of Non-Random Mating:
- Assortative Mating: Individuals with similar phenotypes tend to mate more often than expected by chance. This can lead to an increase in homozygosity (having two identical alleles for a gene) for the traits involved. For example, in plants, self-fertilization is a strong form of assortative mating.
- Disassortative Mating: Individuals with dissimilar phenotypes tend to mate more often than expected by chance. This can lead to an increase in heterozygosity (having two different alleles for a gene) for the traits involved.
Impact on Hardy-Weinberg Equilibrium: Non-random mating patterns alter genotype frequencies without changing allele frequencies. While the overall proportion of alleles remains the same, the distribution of alleles into genotypes changes. This departure from expected genotype frequencies signifies a deviation from Hardy-Weinberg equilibrium.
3. No Gene Flow: Isolation Preserves Equilibrium
Gene flow refers to the movement of alleles between populations. This occurs through the migration of individuals or the dispersal of gametes (reproductive cells). If individuals migrate between populations with different allele frequencies, the recipient population's allele frequencies will change. This influx or efflux of genes disrupts the Hardy-Weinberg equilibrium within the population experiencing the gene flow.
Consequences of Gene Flow: Gene flow can introduce new alleles into a population, increasing genetic diversity. It can also homogenize populations, reducing the genetic differences between them. In the context of the Hardy-Weinberg equilibrium, gene flow acts as a powerful evolutionary force, pushing populations away from equilibrium by altering allele frequencies.
4. No Genetic Drift: The Power of Large Population Size
Genetic drift refers to random fluctuations in allele frequencies that occur solely due to chance events. These fluctuations are especially pronounced in small populations. Imagine a population with a rare allele; if individuals carrying that allele fail to reproduce by random chance, the allele may be lost entirely from the population, regardless of its selective advantage or disadvantage.
Bottleneck Effect and Founder Effect: Two significant examples of genetic drift are the bottleneck effect and the founder effect.
- Bottleneck Effect: A drastic reduction in population size (e.g., due to a natural disaster) can lead to a random loss of alleles, resulting in a significant shift in allele frequencies in the surviving population.
- Founder Effect: When a small group of individuals colonizes a new area, the allele frequencies in the new population may differ significantly from those in the original population simply due to chance.
Impact on Hardy-Weinberg Equilibrium: Genetic drift violates the Hardy-Weinberg equilibrium because allele frequencies change randomly, not due to selective pressures. Large populations are less susceptible to the effects of genetic drift because the random fluctuations in allele frequencies are less impactful.
5. No Natural Selection: Equal Fitness for All Genotypes
Natural selection is the process by which organisms better adapted to their environment tend to survive and reproduce more successfully than less well-adapted organisms. This differential reproductive success leads to changes in allele frequencies within a population. If certain genotypes have higher survival or reproductive rates than others, their alleles will become more common in subsequent generations, directly violating the Hardy-Weinberg equilibrium.
Types of Natural Selection: Different forms of natural selection can lead to various changes in allele frequencies:
- Directional Selection: Favors one extreme phenotype over others.
- Stabilizing Selection: Favors intermediate phenotypes.
- Disruptive Selection: Favors both extreme phenotypes over intermediate phenotypes.
Impact on Hardy-Weinberg Equilibrium: Natural selection is a powerful evolutionary force that consistently drives populations away from Hardy-Weinberg equilibrium. As allele frequencies change due to differential survival and reproduction, the population's genetic makeup evolves.
Conclusion: A Theoretical Ideal and a Powerful Tool
The Hardy-Weinberg principle, despite its idealized conditions, provides a valuable framework for understanding evolution. By comparing observed allele and genotype frequencies in real-world populations to the Hardy-Weinberg expectations, we can identify which evolutionary forces are acting upon those populations. The deviations from equilibrium reveal the interplay of mutation, non-random mating, gene flow, genetic drift, and natural selection, shaping the genetic diversity and evolution of life on Earth. Understanding these five conditions is key to unraveling the complex tapestry of evolutionary processes.
Latest Posts
Latest Posts
-
A Covalent Bond Is Formed As A Result Of
Apr 05, 2025
-
What Is A Net Change In Math
Apr 05, 2025
-
Is Corrosion A Physical Or Chemical Property
Apr 05, 2025
-
Find The Equation Of The Tangent Plane To The Surface
Apr 05, 2025
-
Third Trophic Level In The Food Chain
Apr 05, 2025
Related Post
Thank you for visiting our website which covers about 5 Conditions Of Hardy Weinberg Equilibrium . We hope the information provided has been useful to you. Feel free to contact us if you have any questions or need further assistance. See you next time and don't miss to bookmark.