Describe The Role Of Carbon In Biological Systems.
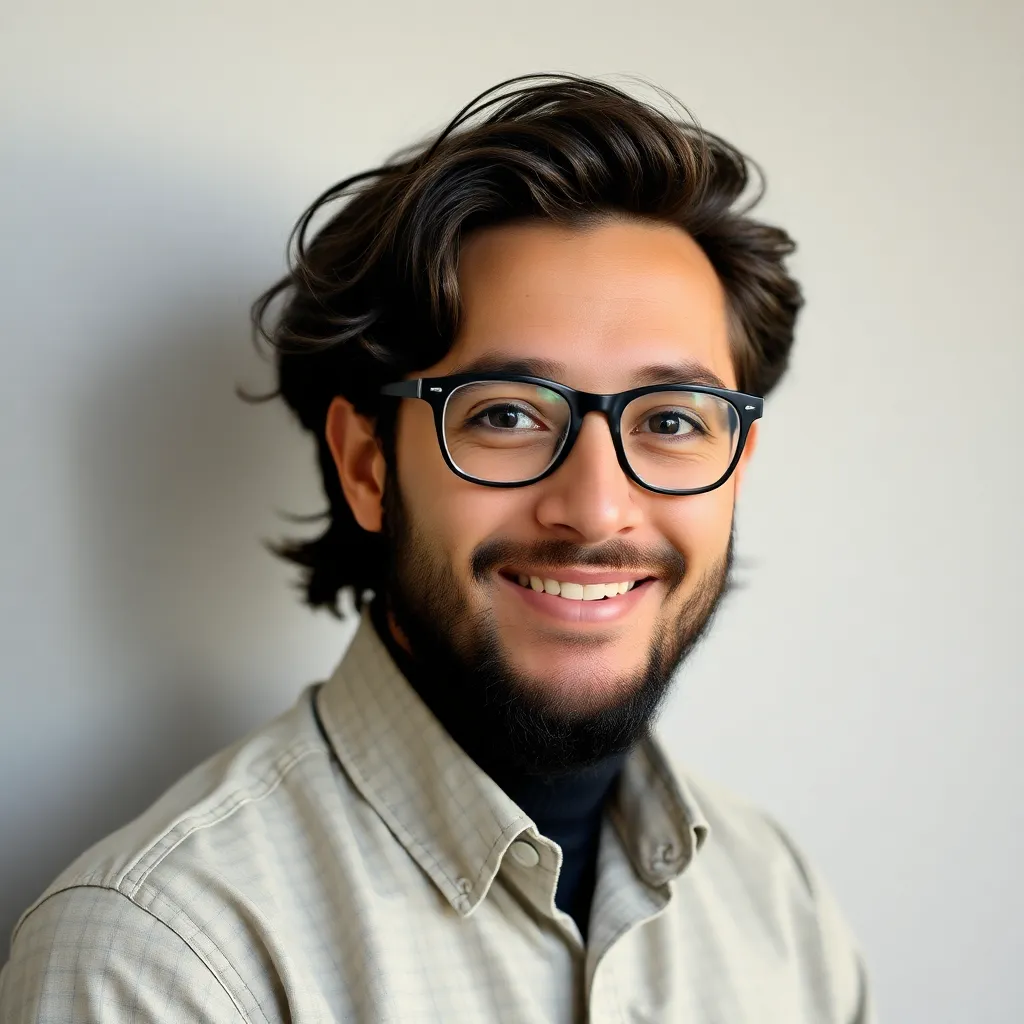
Muz Play
Apr 01, 2025 · 6 min read
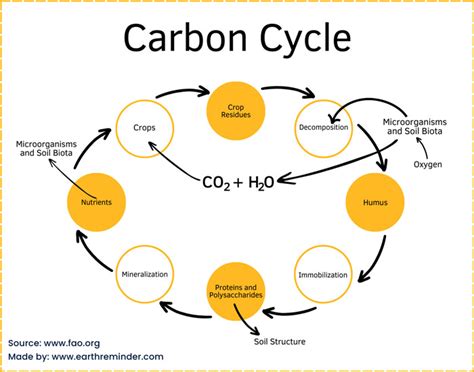
Table of Contents
- Describe The Role Of Carbon In Biological Systems.
- Table of Contents
- The Indispensable Role of Carbon in Biological Systems
- The Unique Properties of Carbon
- 1. Tetravalency:
- 2. Catination:
- 3. Isomerism:
- Carbon in Biomolecules: The Building Blocks of Life
- 1. Carbohydrates: Energy and Structure
- 2. Lipids: Energy Storage and Membranes
- 3. Proteins: Workhorses of the Cell
- 4. Nucleic Acids: Information Carriers
- The Carbon Cycle: A Global Perspective
- Key Processes in the Carbon Cycle:
- Disruptions to the Carbon Cycle and Their Implications
- Consequences of Carbon Cycle Disruption:
- Conclusion: The Future of Carbon in Biological Systems
- Latest Posts
- Latest Posts
- Related Post
The Indispensable Role of Carbon in Biological Systems
Carbon, the sixth element on the periodic table, is the cornerstone of life as we know it. Its unique properties make it the ideal building block for the incredibly diverse and complex molecules that constitute all living organisms. Without carbon, life as we understand it simply wouldn't exist. This article will delve deep into the multifaceted role of carbon in biological systems, exploring its presence in various biomolecules, its cycling through the environment, and the implications of its disruption.
The Unique Properties of Carbon
Carbon's central role stems from its exceptional chemical versatility. This versatility arises from several key properties:
1. Tetravalency:
Carbon possesses four valence electrons, allowing it to form four strong covalent bonds with other atoms, including other carbon atoms. This capacity for multiple bonding enables the formation of long chains, branched structures, and rings, leading to the vast array of organic molecules. This tetravalency is unparalleled amongst other elements, enabling the creation of the intricate frameworks of biological macromolecules.
2. Catination:
Carbon atoms exhibit a remarkable ability to bond with other carbon atoms, forming chains and rings of virtually unlimited length. This property, known as catenation, is crucial for building the complex structures of carbohydrates, lipids, proteins, and nucleic acids – the four fundamental classes of biomolecules. The strength and stability of carbon-carbon bonds ensure the structural integrity of these essential molecules.
3. Isomerism:
The capacity of carbon to form diverse structures leads to the phenomenon of isomerism. Isomers are molecules with the same chemical formula but different structural arrangements. This allows for a vast diversity of molecules with unique properties, even with the same basic carbon framework. This is critical for the specificity of biological interactions and the functional diversity of biomolecules.
Carbon in Biomolecules: The Building Blocks of Life
Carbon acts as the structural backbone for all major classes of biological molecules. Let's examine its contribution to each:
1. Carbohydrates: Energy and Structure
Carbohydrates, composed of carbon, hydrogen, and oxygen, are essential for energy storage and structural support. Simple sugars like glucose (C₆H₁₂O₆) are fundamental energy sources. More complex carbohydrates, such as starch and cellulose, are polymers of glucose units linked together through carbon-carbon and carbon-oxygen bonds. Starch serves as an energy reserve in plants, while cellulose provides structural rigidity in plant cell walls. The intricate structures and diverse functionalities of carbohydrates are directly dependent on carbon's bonding capabilities.
2. Lipids: Energy Storage and Membranes
Lipids, including fats and oils, are primarily composed of carbon, hydrogen, and oxygen. They are crucial for energy storage, membrane structure, and hormone synthesis. The long hydrocarbon chains in fatty acids are fundamentally carbon chains, with hydrogen atoms attached. The diverse structures of lipids, from triglycerides to phospholipids, are based on variations in these carbon chains and the functional groups attached to them. The hydrophobic nature of the hydrocarbon chains, a consequence of carbon's nonpolarity, is vital for membrane formation and function.
3. Proteins: Workhorses of the Cell
Proteins are complex polymers of amino acids, linked together by peptide bonds. Each amino acid contains a central carbon atom (the α-carbon) bonded to an amino group, a carboxyl group, a hydrogen atom, and a side chain (R-group). The R-group determines the unique properties of each amino acid. The sequence and arrangement of amino acids, dictated by the carbon backbone, determine the three-dimensional structure and function of the protein. Proteins are involved in virtually every aspect of cellular function, acting as enzymes, structural components, transporters, and signaling molecules. Their elaborate structures are a testament to carbon's versatility.
4. Nucleic Acids: Information Carriers
Nucleic acids, DNA and RNA, are responsible for storing and transmitting genetic information. Their building blocks, nucleotides, contain a sugar (ribose in RNA, deoxyribose in DNA), a phosphate group, and a nitrogenous base. The sugar molecules, with their carbon-oxygen backbones, are crucial for the structural integrity of the nucleic acid polymers. The sequence of nitrogenous bases, attached to the carbon atoms in the sugar molecules, encodes the genetic instructions. The double helix structure of DNA, the foundation of heredity, depends on the precise arrangement of carbon atoms and their interactions with other atoms within the molecule.
The Carbon Cycle: A Global Perspective
Carbon is not static; it cycles through the biosphere, geosphere, atmosphere, and hydrosphere in a complex and dynamic process known as the carbon cycle. This cycle is crucial for maintaining the balance of life on Earth.
Key Processes in the Carbon Cycle:
-
Photosynthesis: Plants and other photosynthetic organisms absorb atmospheric carbon dioxide (CO₂) and use it to synthesize organic molecules, such as glucose, during photosynthesis. This process effectively removes carbon from the atmosphere and incorporates it into living biomass.
-
Respiration: All living organisms, including plants and animals, release carbon dioxide into the atmosphere through respiration. This is the process by which organisms break down organic molecules to generate energy.
-
Decomposition: Decomposers, such as bacteria and fungi, break down dead organic matter, releasing carbon dioxide back into the atmosphere or into the soil as organic carbon.
-
Ocean Uptake: The oceans absorb significant amounts of atmospheric carbon dioxide, forming carbonic acid and bicarbonate ions. This ocean uptake plays a critical role in regulating atmospheric CO₂ levels.
-
Sedimentation: Carbon can be stored in sediments over geological timescales, eventually forming fossil fuels such as coal, oil, and natural gas.
Disruptions to the Carbon Cycle and Their Implications
Human activities, particularly the burning of fossil fuels and deforestation, have significantly disrupted the natural carbon cycle. This disruption leads to an increased concentration of atmospheric CO₂, which is the primary driver of climate change.
Consequences of Carbon Cycle Disruption:
-
Climate Change: The increased atmospheric CO₂ concentration leads to global warming, causing a cascade of effects, including rising sea levels, more frequent and intense extreme weather events, and disruptions to ecosystems.
-
Ocean Acidification: Increased atmospheric CO₂ leads to ocean acidification as the ocean absorbs more CO₂. This acidification threatens marine organisms with calcium carbonate shells or skeletons, such as corals and shellfish.
-
Ecosystem Disruptions: Changes in temperature and precipitation patterns caused by climate change disrupt ecosystems, impacting biodiversity and the provision of ecosystem services.
-
Human Health Impacts: Climate change impacts human health through increased heat stress, the spread of infectious diseases, and disruptions to food security and water resources.
Conclusion: The Future of Carbon in Biological Systems
Carbon's role in biological systems is fundamental and multifaceted. Its unique properties enable the creation of the complex molecules that underpin life. Understanding the carbon cycle and the impacts of human activities on this cycle is crucial for addressing the challenges of climate change and preserving the health of our planet. Continued research into carbon sequestration, renewable energy sources, and sustainable practices is essential for mitigating the negative impacts of carbon cycle disruption and ensuring the future of life on Earth. The future of our planet hinges on our ability to manage and understand the intricate role of carbon in the delicate balance of biological systems. As we move forward, fostering a deeper understanding of carbon's role, both within organisms and on a global scale, will be paramount to our continued survival and the prosperity of future generations. This requires a collaborative effort spanning scientific research, technological innovation, and policy changes to ensure a sustainable and carbon-conscious future.
Latest Posts
Latest Posts
-
Difference Between Basic Science And Applied Science
Apr 05, 2025
-
The Atp Made During Glycolysis Is Generated By
Apr 05, 2025
-
Term Used For Compounds With Properties For Neutralizing Acids
Apr 05, 2025
-
How To Find Average Acceleration From Velocity Time Graph
Apr 05, 2025
-
Newtons Second Law In Rotational Form
Apr 05, 2025
Related Post
Thank you for visiting our website which covers about Describe The Role Of Carbon In Biological Systems. . We hope the information provided has been useful to you. Feel free to contact us if you have any questions or need further assistance. See you next time and don't miss to bookmark.