How Does Molecular Biology Support The Theory Of Evolution
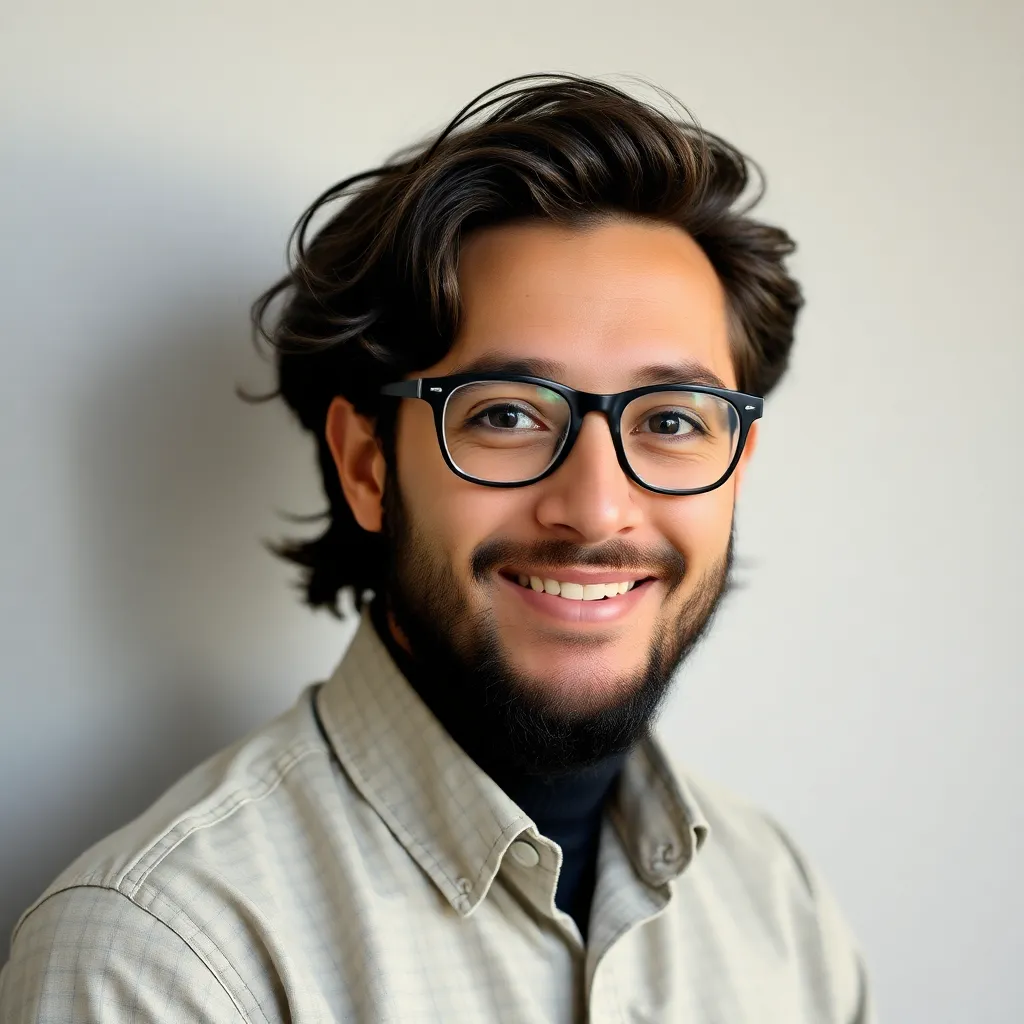
Muz Play
Apr 03, 2025 · 6 min read
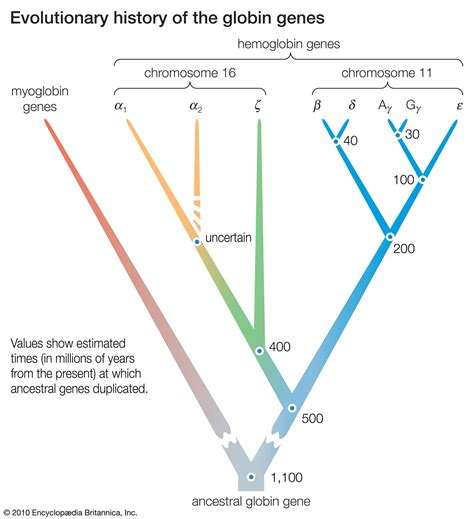
Table of Contents
How Molecular Biology Supports the Theory of Evolution
The theory of evolution, the unifying concept of biology, posits that life on Earth has changed over time through a process of descent with modification. While Darwin and Wallace laid the groundwork for this theory based on observable traits and fossil evidence, molecular biology has provided a powerful arsenal of evidence that has solidified and refined our understanding of evolutionary processes. This article delves into the multifaceted ways molecular biology supports the theory of evolution, providing compelling evidence from various molecular levels.
The Molecular Clock: Tracking Evolutionary Time
One of the most compelling contributions of molecular biology to evolutionary theory is the concept of the molecular clock. This concept utilizes the accumulation of mutations in DNA and protein sequences over time as a measure of evolutionary divergence. The basic principle is that mutations occur at a relatively constant rate in certain genes, allowing scientists to estimate the time elapsed since two species diverged from a common ancestor. This is analogous to using the ticking of a clock to measure time, hence the name "molecular clock."
Calibration and Limitations:
While the molecular clock provides a powerful tool, it's crucial to understand its limitations. The rate of mutation can vary depending on factors such as generation time, environmental influences, and the specific gene being studied. Therefore, calibrating the clock requires careful consideration of these factors, often using independent fossil evidence or other dating methods. Despite these limitations, the molecular clock has proven remarkably useful in reconstructing phylogenetic trees and estimating divergence times for a wide range of organisms.
Mitochondrial DNA and the Molecular Clock:
Mitochondrial DNA (mtDNA), a small circular chromosome found in mitochondria, has been particularly useful in molecular clock studies. mtDNA has a high mutation rate compared to nuclear DNA and lacks recombination (shuffling of genetic material during sexual reproduction), simplifying the analysis. This makes it a valuable tool for tracing evolutionary relationships, particularly in closely related species. For example, studies using mtDNA have shed light on the evolutionary history of humans and other primates, offering insights into migration patterns and population diversification.
Homologous Genes and Shared Ancestry:
The presence of homologous genes across diverse species provides strong support for common ancestry. Homologous genes are genes that are derived from a common ancestor and share a significant degree of sequence similarity. The degree of similarity reflects the evolutionary distance between species; closely related species have more similar homologous genes than distantly related species.
Orthologs and Paralogs:
Within the realm of homologous genes, two key categories exist: orthologs and paralogs. Orthologs are homologous genes found in different species that arose from a common ancestor through speciation. Paralogs, on the other hand, are homologous genes found within the same species that arose through gene duplication. Both orthologs and paralogs provide valuable insights into evolutionary relationships, with orthologs reflecting the evolutionary history of species and paralogs shedding light on the evolution of gene functions within a species.
Gene Families and Functional Diversification:
The study of gene families, groups of homologous genes with related functions, reveals how genes evolve and diversify over time. Gene duplication followed by mutation and selection can lead to the emergence of new gene functions, providing raw material for evolutionary innovation. This process is clearly evident in the evolution of globin genes, which have diversified to perform oxygen transport in different tissues and developmental stages.
Pseudogenes: Vestiges of Evolutionary History:
Pseudogenes, non-functional copies of genes, represent another compelling line of molecular evidence supporting evolution. Pseudogenes arise from gene duplication or retrotransposition events, but unlike functional genes, they lose their function over time due to accumulated mutations. Their presence in the genome is a testament to the evolutionary history of the organism, revealing past gene function and providing insights into gene inactivation processes. The existence of pseudogenes is difficult to reconcile with creationist viewpoints, as they are non-functional genetic elements that carry no apparent benefit.
Comparative Genomics: Unveiling Evolutionary Relationships:
The field of comparative genomics, which compares the genomes of different species, has revolutionized our understanding of evolutionary relationships. By comparing the entire genomes of different species, researchers can identify homologous genes, conserved genomic regions, and other molecular features that reflect evolutionary history. This data is used to construct phylogenetic trees, illustrating the evolutionary relationships between organisms and providing a framework for understanding the diversification of life.
Genome Size and Complexity:
Comparative genomics has revealed that genome size does not always correlate with organismal complexity. Some organisms with relatively small genomes (e.g., some bacteria) have intricate lifestyles and complex interactions with their environment, while some organisms with very large genomes (e.g., some plants and amphibians) have simpler lifestyles. This observation contradicts the notion that increased genome size automatically equates to greater complexity and supports the evolutionary view that complexity arises through various mechanisms, including gene regulation and other non-coding elements.
Molecular Phylogenetics: Constructing the Tree of Life:
Molecular phylogenetics uses molecular data, such as DNA and protein sequences, to reconstruct the evolutionary relationships between organisms. By comparing the sequences of homologous genes across different species, scientists can infer phylogenetic relationships and build phylogenetic trees, which depict the evolutionary history of a group of organisms. These trees frequently align with those built from morphological characteristics, strengthening the evidence for common ancestry.
Phylogenetic Trees and Evolutionary Relationships:
Phylogenetic trees are visual representations of evolutionary relationships, with branch points indicating divergence events and branch lengths representing evolutionary time or genetic distance. Molecular phylogenetic analyses have revolutionized taxonomy and our understanding of evolutionary history, revealing unexpected relationships between organisms and challenging previously held classifications.
Evolution of Development (Evo-Devo): Molecular Insights into Form and Function:
Evo-devo, a field that integrates evolutionary biology and developmental biology, studies how changes in developmental genes and pathways drive evolutionary changes in morphology and physiology. Molecular biology has played a crucial role in understanding the role of homeobox (Hox) genes, master regulatory genes that control the body plan during development. Hox genes are remarkably conserved across diverse animal groups, providing compelling evidence for their ancient origins and shared ancestry. However, changes in the expression patterns or sequences of Hox genes can lead to significant evolutionary changes in body plan.
The Power of Small Changes:
Evo-devo highlights that relatively small changes in gene regulation or expression can have dramatic effects on the phenotype, demonstrating the power of developmental mechanisms in driving evolutionary change. This finding underscores the role of regulatory mutations in the evolution of morphological diversity, a point not extensively considered in early evolutionary theories.
Horizontal Gene Transfer and the Evolution of Prokaryotes:
Horizontal gene transfer (HGT), the movement of genetic material between organisms other than through vertical inheritance (parent to offspring), is particularly significant in the evolution of prokaryotes. HGT plays a crucial role in bacterial adaptation and evolution, facilitating the rapid spread of antibiotic resistance and other traits. While less common in eukaryotes, HGT still occurs and can have significant evolutionary implications. The existence of HGT further demonstrates the dynamic and flexible nature of evolution.
Conclusion:
Molecular biology has provided an unprecedented wealth of evidence supporting the theory of evolution. From the molecular clock to comparative genomics and evo-devo, molecular data has deepened our understanding of evolutionary processes, refining evolutionary trees and revealing the underlying mechanisms driving evolutionary change. The convergence of molecular evidence with fossil evidence, comparative anatomy, and biogeography strongly supports the theory of evolution as the unifying framework for understanding life's diversity on Earth. The ongoing advancements in molecular biology promise to further refine and expand our understanding of the intricate tapestry of life's history.
Latest Posts
Latest Posts
-
What Is Steric Hindrance In Organic Chemistry
Apr 04, 2025
-
Why Are Seeds An Evolutionary Advantage For Seed Plants
Apr 04, 2025
-
Do Cations Lose Or Gain Electrons
Apr 04, 2025
-
Most Likely Cations And Anions On The Periodic Table
Apr 04, 2025
-
What Is Feedback Inhibition In Biology
Apr 04, 2025
Related Post
Thank you for visiting our website which covers about How Does Molecular Biology Support The Theory Of Evolution . We hope the information provided has been useful to you. Feel free to contact us if you have any questions or need further assistance. See you next time and don't miss to bookmark.