Predicting Acid Or Base Strength From The Conjugate
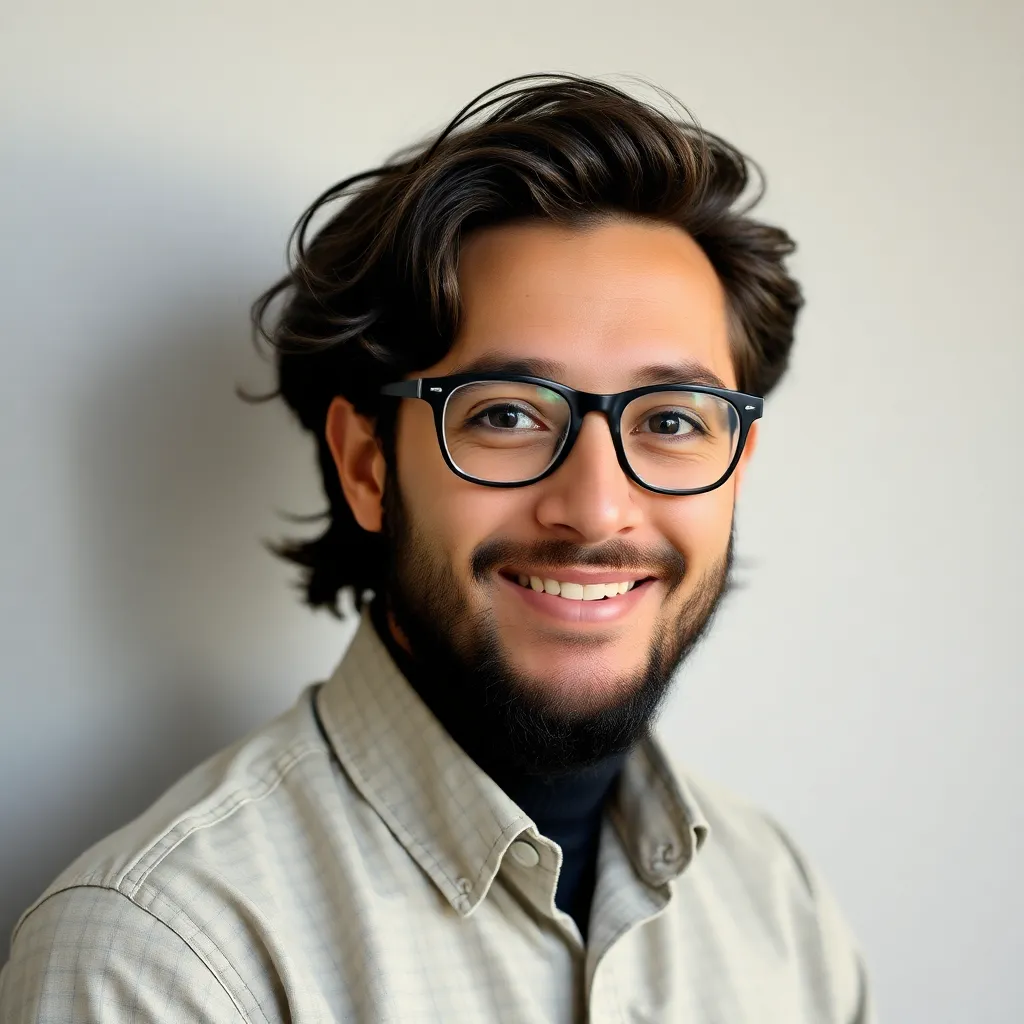
Muz Play
Mar 29, 2025 · 7 min read
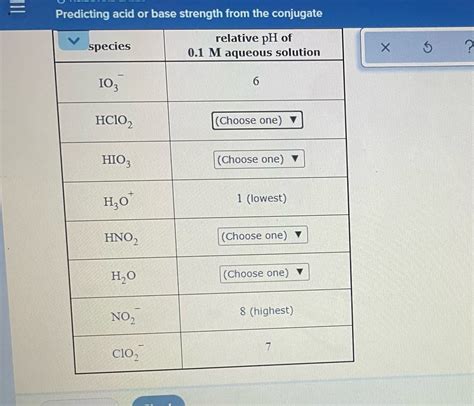
Table of Contents
Predicting Acid or Base Strength from the Conjugate: A Comprehensive Guide
Understanding acid and base strength is fundamental in chemistry. While memorizing pKa values is helpful, predicting relative strength based on the structure of the conjugate acid or base offers a deeper, more insightful approach. This ability is crucial for various applications, from designing new pharmaceuticals to understanding biochemical processes. This comprehensive guide delves into the principles and strategies for accurately predicting the strength of acids and bases based on their conjugates.
The Conjugate Acid-Base Pair: A Foundation
Before diving into prediction methods, let's establish a firm grasp of the conjugate acid-base pair concept. According to the Brønsted-Lowry theory, an acid is a proton (H⁺) donor, while a base is a proton acceptor. When an acid donates a proton, it forms its conjugate base. Conversely, when a base accepts a proton, it forms its conjugate acid.
The strength of an acid is inversely related to the strength of its conjugate base, and vice versa. A strong acid will have a weak conjugate base, and a weak acid will have a strong conjugate base. This relationship is a cornerstone of our predictive strategies.
Understanding pKa and pKb Values
The pKa value is a measure of acid strength, representing the negative logarithm of the acid dissociation constant (Ka). A lower pKa value indicates a stronger acid. Similarly, the pKb value measures base strength, representing the negative logarithm of the base dissociation constant (Kb). A lower pKb value signifies a stronger base. Crucially, pKa and pKb are related by the equation:
pKa + pKb = 14 (at 25°C)
This relationship allows us to determine the strength of a base if we know the pKa of its conjugate acid, and vice-versa.
Predicting Acid Strength from the Conjugate Base
Predicting the acid strength from its conjugate base relies on analyzing the stability of the conjugate base. A more stable conjugate base implies a stronger acid. Several factors influence conjugate base stability:
1. Resonance Stabilization:
Resonance significantly impacts conjugate base stability. If the negative charge (or positive charge in the case of conjugate acids of weak bases) on the conjugate base can be delocalized across multiple atoms through resonance, the stability increases, making the parent acid stronger. For example, compare acetic acid (CH₃COOH) and its conjugate base (CH₃COO⁻). The negative charge on the acetate ion is delocalized across two oxygen atoms through resonance, making it relatively stable and acetic acid a weak acid.
Example: Compare the acidity of phenol (C₆H₅OH) and ethanol (CH₃CH₂OH). The phenoxide ion (conjugate base of phenol) benefits from resonance stabilization, distributing the negative charge across the aromatic ring. The ethoxide ion (conjugate base of ethanol) lacks this resonance stabilization. Therefore, phenol is a significantly stronger acid than ethanol.
2. Inductive Effects:
Electron-withdrawing groups (EWGs) stabilize negative charges, while electron-donating groups (EDGs) destabilize them. The presence of EWGs near the negatively charged atom in the conjugate base increases its stability, making the parent acid stronger. Conversely, EDGs decrease stability, weakening the acid.
Example: Compare the acidity of trifluoroacetic acid (CF₃COOH) and acetic acid (CH₃COOH). The three fluorine atoms in trifluoroacetic acid are highly electronegative EWGs. They pull electron density away from the carboxylate group (conjugate base), stabilizing the negative charge and making trifluoroacetic acid a much stronger acid than acetic acid.
3. Hybridization:
The hybridization of the atom bearing the negative charge also affects stability. A more electronegative atom can better accommodate the negative charge. Sp hybridized carbons are more electronegative than sp² or sp³ hybridized carbons. Similarly, sp² hybridized carbons are more electronegative than sp³ hybridized carbons. Therefore, anionic conjugate bases on sp hybridized carbons are more stable, making the parent acid stronger.
Example: Acetylene (HC≡CH) is a much weaker acid than ethene (H₂C=CH₂) or ethane (H₃C-CH₃). The conjugate base of acetylene (HC≡C⁻) has the negative charge on an sp hybridized carbon, making it more stable than the conjugate bases of ethene (CH₂=CH⁻) and ethane (CH₃CH₂⁻), where the negative charge is on sp² and sp³ hybridized carbons, respectively.
4. Size and Polarizability:
Larger atoms and anions are generally more polarizable. This means their electron clouds can be distorted more easily, accommodating the negative charge more effectively and increasing stability. Larger conjugate bases tend to be more stable, resulting in stronger parent acids.
Example: Compare the acidity of HI, HBr, and HCl. The iodide ion (I⁻) is the largest and most polarizable, making it the most stable conjugate base and HI the strongest acid.
5. Aromaticity:
If the conjugate base is aromatic, it gains extra stability due to the delocalization of pi electrons within the ring system. This significantly enhances the stability of the conjugate base and consequently, increases the acidity of the parent acid.
Predicting Base Strength from the Conjugate Acid
Predicting base strength from the conjugate acid follows a similar logic but in reverse. A weaker conjugate acid suggests a stronger base. Factors influencing conjugate acid stability mirror those affecting conjugate base stability, but with opposite effects:
1. Resonance Destabilization in Conjugate Acids:
Resonance can destabilize the conjugate acid if it leads to placing a positive charge on an electronegative atom.
2. Inductive Effects in Conjugate Acids:
Electron-donating groups (EDGs) destabilize positive charges in conjugate acids, whereas electron-withdrawing groups (EWGs) stabilize them.
3. Hybridization in Conjugate Acids:
The hybridization state of the atom carrying the positive charge also plays a crucial role. Less electronegative atoms are less capable of carrying a positive charge.
4. Size and Polarizability in Conjugate Acids:
Larger atoms are less effective in supporting positive charges due to lower charge density.
5. Aromaticity in Conjugate Acids:
Aromatic conjugate acids are generally less stable due to the disruption of aromaticity when the proton is added.
Practical Applications and Examples
The principles discussed above have wide-ranging applications:
-
Drug Design: Understanding the acidity/basicity of functional groups in drug molecules is critical for predicting their absorption, distribution, metabolism, and excretion (ADME) properties.
-
Catalysis: Many catalysts function through acid-base reactions. Predicting acid or base strength helps design efficient catalysts.
-
Environmental Chemistry: Understanding the acidity of pollutants like acid rain is vital for assessing environmental impact.
-
Biochemical Processes: Many biological processes involve acid-base reactions, and understanding their strengths is essential for studying these processes.
Example 1: Comparing the Acidity of Carboxylic Acids and Alcohols:
Carboxylic acids are generally stronger acids than alcohols. This is because the conjugate base of a carboxylic acid (carboxylate ion) is resonance-stabilized, while the conjugate base of an alcohol (alkoxide ion) is not.
Example 2: Comparing the Basicity of Amines:
Amines (R-NH₂) are bases. Their basicity is affected by inductive effects. Electron-donating groups increase basicity, while electron-withdrawing groups decrease it. For example, aniline (C₆H₅NH₂) is a weaker base than methylamine (CH₃NH₂) because the phenyl group is an electron-withdrawing group.
Example 3: Predicting the Acidity of a New Compound:
Imagine a new molecule containing a hydroxyl group (-OH) attached to a benzene ring with a nitro group (-NO₂) as a substituent. The nitro group is a strong electron-withdrawing group. Therefore, we predict that this molecule will be a stronger acid than phenol (C₆H₅OH) due to the increased stabilization of the conjugate base by the nitro group.
Advanced Considerations
Predicting acid and base strength isn't always straightforward. Steric effects, solvation effects, and other factors can influence the overall strength. These advanced considerations often require sophisticated computational methods for accurate predictions.
Conclusion
Predicting acid and base strength from their conjugates offers a powerful tool for understanding and manipulating chemical reactions. By carefully analyzing the stability of the conjugate acid or base based on resonance, inductive effects, hybridization, size, and aromaticity, one can make accurate predictions about relative acid and base strengths. This knowledge is invaluable in diverse fields, from drug discovery to environmental science. While simple rules of thumb exist, remember that more complex scenarios may require more sophisticated theoretical and computational approaches for accurate predictions. Continuous learning and the development of refined predictive models remain crucial to advancing our understanding of acid-base chemistry.
Latest Posts
Latest Posts
-
How Many Sides Does A Parallelogram Have
Mar 31, 2025
-
Elements That Are Gases At Room Temperature
Mar 31, 2025
-
How Did Abraham Lincolns Assassination Affect Reconstruction
Mar 31, 2025
-
How To Construct A Standard Curve On Excel
Mar 31, 2025
-
Does A Gas Have A Fixed Volume
Mar 31, 2025
Related Post
Thank you for visiting our website which covers about Predicting Acid Or Base Strength From The Conjugate . We hope the information provided has been useful to you. Feel free to contact us if you have any questions or need further assistance. See you next time and don't miss to bookmark.