What Happens To Electrons In Metallic Bonding
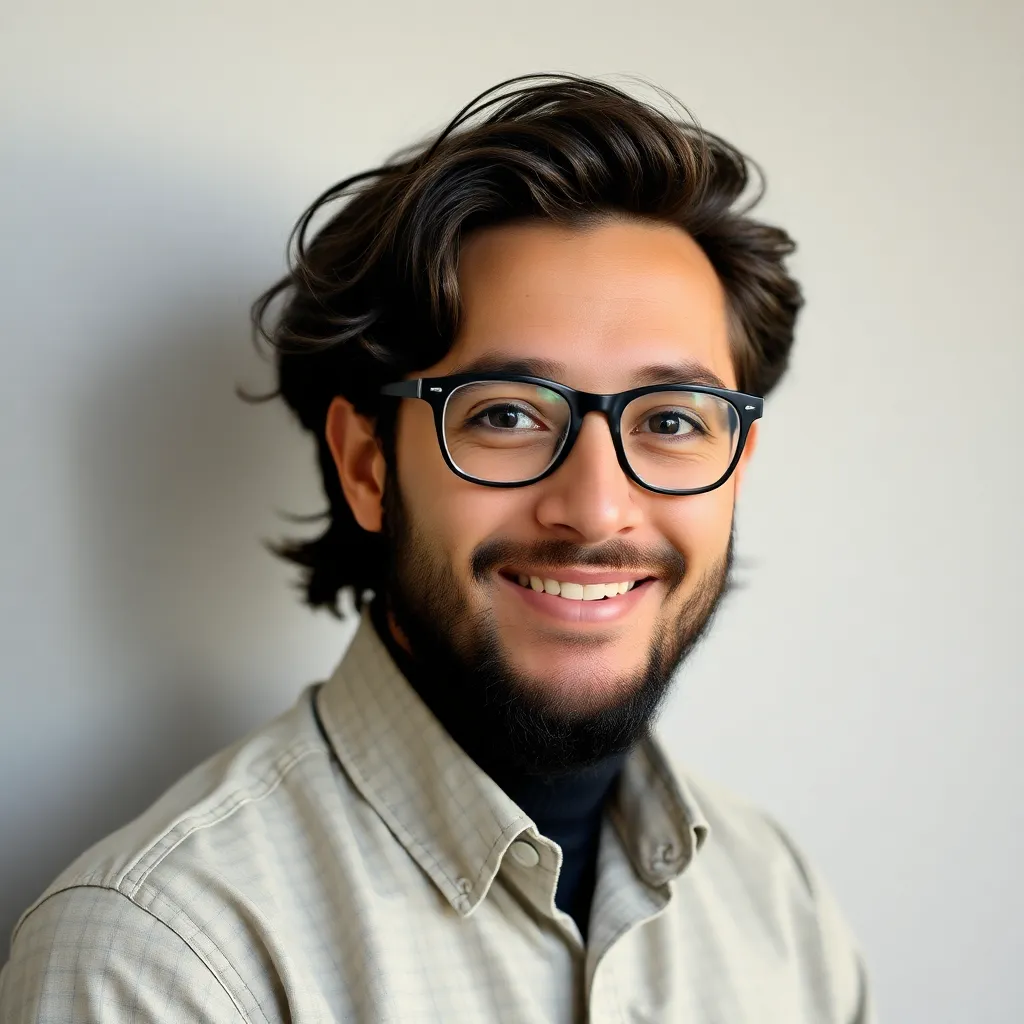
Muz Play
Mar 26, 2025 · 6 min read
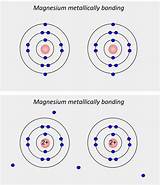
Table of Contents
What Happens to Electrons in Metallic Bonding? Delving into the Sea of Electrons
Metallic bonding is a fascinating phenomenon responsible for many of the unique properties we associate with metals: their conductivity, malleability, ductility, and lustrous appearance. Understanding what happens to electrons in metallic bonding is key to understanding these properties. This article dives deep into the intricacies of this bonding type, exploring the "sea of electrons" model, the implications for electrical and thermal conductivity, and the influence on the mechanical properties of metals.
The Sea of Electrons Model: A Foundation for Understanding
Unlike covalent or ionic bonding where electrons are localized between specific atoms or transferred completely, metallic bonding involves a unique delocalized electron arrangement. In a metal, the valence electrons—the outermost electrons in an atom—are not associated with any particular atom. Instead, they are free to move throughout the entire metallic structure. This creates a "sea" or "cloud" of delocalized electrons that are shared collectively by all the metal atoms in the lattice.
Visualizing the Sea: A Simple Analogy
Imagine a lattice of positively charged metal ions (cations) submerged in a "sea" of negatively charged, freely moving electrons. The strong electrostatic attraction between these positive ions and the electron sea holds the structure together. The electrons are not tightly bound to individual atoms; they're constantly in motion, flowing through the spaces between the ions. This constant movement is crucial to understanding the metal's properties.
The Role of Valence Electrons
The number of valence electrons an atom possesses significantly impacts the strength of the metallic bond. Metals with more valence electrons generally form stronger metallic bonds because there are more electrons contributing to the electron sea, increasing the electrostatic attraction with the positive ions. This translates into higher melting and boiling points. For example, transition metals, with multiple valence electrons, often exhibit higher melting points than alkali metals with only one valence electron.
Conductivity: The Hallmark of Metallic Bonding
The "sea of electrons" model elegantly explains the exceptional electrical and thermal conductivity of metals.
Electrical Conductivity: A Flow of Charge
When an electric field is applied across a metal, the delocalized electrons are easily mobilized. They can readily move in response to the field, creating a flow of charge, thus leading to high electrical conductivity. This is in stark contrast to ionic or covalent compounds where electron movement is significantly restricted. The ease with which these electrons move is why metals are excellent conductors of electricity.
Thermal Conductivity: Transferring Energy
Similarly, the free movement of electrons facilitates efficient thermal conductivity. When one part of a metal is heated, the increased kinetic energy of the electrons is rapidly transferred throughout the metal via collisions with other electrons and the metal ions. This rapid energy transfer contributes to the metal's high thermal conductivity. The more freely electrons can move, the more efficiently heat is transferred through the material.
Malleability and Ductility: Shaping Metals
Metals are known for their ability to be deformed without breaking – properties known as malleability (the ability to be hammered into sheets) and ductility (the ability to be drawn into wires). The sea of electrons plays a crucial role in these mechanical properties.
Shifting Ions, Maintaining Bonds
When a metal is subjected to stress, such as hammering or stretching, the layers of metal ions can slide past one another. Because the electron sea is delocalized, the metallic bonding is not disrupted by this movement. The electron sea acts as a "glue," maintaining the electrostatic attraction between the metal ions even as they shift positions. This allows metals to deform extensively before breaking. This is unlike ionic compounds, where the rigid structure and localized charges make them brittle.
Dislocations and Plastic Deformation
The process of deformation in metals is not perfectly smooth; it often involves imperfections in the crystal lattice called dislocations. These dislocations act as slip planes, facilitating the movement of ions and contributing to the plasticity of metals. The presence of the delocalized electrons allows these dislocations to move relatively easily, enhancing the malleability and ductility.
Lustrous Appearance: The Interaction with Light
The shiny, lustrous appearance of metals is also a direct consequence of the electron sea.
Reflecting Light: Electrons at Work
When light interacts with the surface of a metal, the delocalized electrons readily absorb and re-emit the light. This absorption and re-emission of light across a broad spectrum of wavelengths leads to the characteristic metallic luster. The electrons' ability to interact with light is a consequence of their delocalized nature and free movement. The luster is enhanced by the smooth surface of a polished metal which efficiently reflects the light.
Alloys: Modifying Properties through Metallic Bonding
Alloys are mixtures of two or more metals, often created to modify the properties of the constituent metals. The principles of metallic bonding are central to understanding how alloys behave.
Modifying the Electron Sea
The addition of different metals to an alloy alters the composition and density of the electron sea. This change can significantly impact the properties of the alloy, such as its strength, hardness, and corrosion resistance. For example, adding carbon to iron produces steel, an alloy significantly stronger and harder than pure iron. This strengthening is partly due to the way carbon atoms interact with the electron sea and the iron lattice.
Substitutional and Interstitial Alloys
Two common types of alloys are substitutional and interstitial alloys. In substitutional alloys, atoms of one metal replace atoms of another in the crystal lattice. In interstitial alloys, smaller atoms fit into the spaces between the larger atoms in the lattice. Both types of alloys modify the electron sea and affect the overall properties.
Beyond the Simple Model: Refinements and Complexities
While the sea of electrons model provides a good basic understanding of metallic bonding, it's important to acknowledge its limitations. Real metals are complex systems with various factors affecting their behavior.
Band Theory: A More Sophisticated Approach
A more rigorous theoretical treatment of metallic bonding involves band theory. Band theory considers the interaction of atomic orbitals to form energy bands. In metals, the valence orbitals overlap significantly, forming a continuous band of energy levels that are only partially filled with electrons. This partially filled band allows electrons to move freely through the material, supporting the conductivity observations.
The Influence of Crystal Structure
The crystal structure of a metal also impacts its properties. Different crystal structures (e.g., body-centered cubic, face-centered cubic) influence the arrangement of atoms and the interactions within the electron sea, ultimately affecting the material's mechanical and electrical properties.
Conclusion: A Dynamic and Essential Bonding Type
Metallic bonding is a powerful bonding mechanism responsible for the unique and valuable properties that have made metals essential materials throughout human history. The "sea of electrons" model, while simplified, captures the essence of this bonding type, explaining the excellent conductivity, malleability, ductility, and lustrous appearance of metals. A deeper understanding, incorporating band theory and considering the complexities of crystal structures, provides a more complete picture of this fundamental interaction. The ability to manipulate the electron sea through alloying further enhances the versatility and importance of metals in various technological applications. The ongoing research into metallic bonding continues to unveil new insights, driving innovation and development across various fields of science and engineering.
Latest Posts
Latest Posts
-
How To Find Point Of Tangency
Mar 29, 2025
-
How Do You Calculate Potential Difference
Mar 29, 2025
-
How To Round To Four Decimal Places
Mar 29, 2025
-
Reaction Of Benzoic Acid And Naoh
Mar 29, 2025
-
What Type Of Compounds Dissolve To Become Electrolyte
Mar 29, 2025
Related Post
Thank you for visiting our website which covers about What Happens To Electrons In Metallic Bonding . We hope the information provided has been useful to you. Feel free to contact us if you have any questions or need further assistance. See you next time and don't miss to bookmark.