What Is The Vsepr Geometry Of The Particle
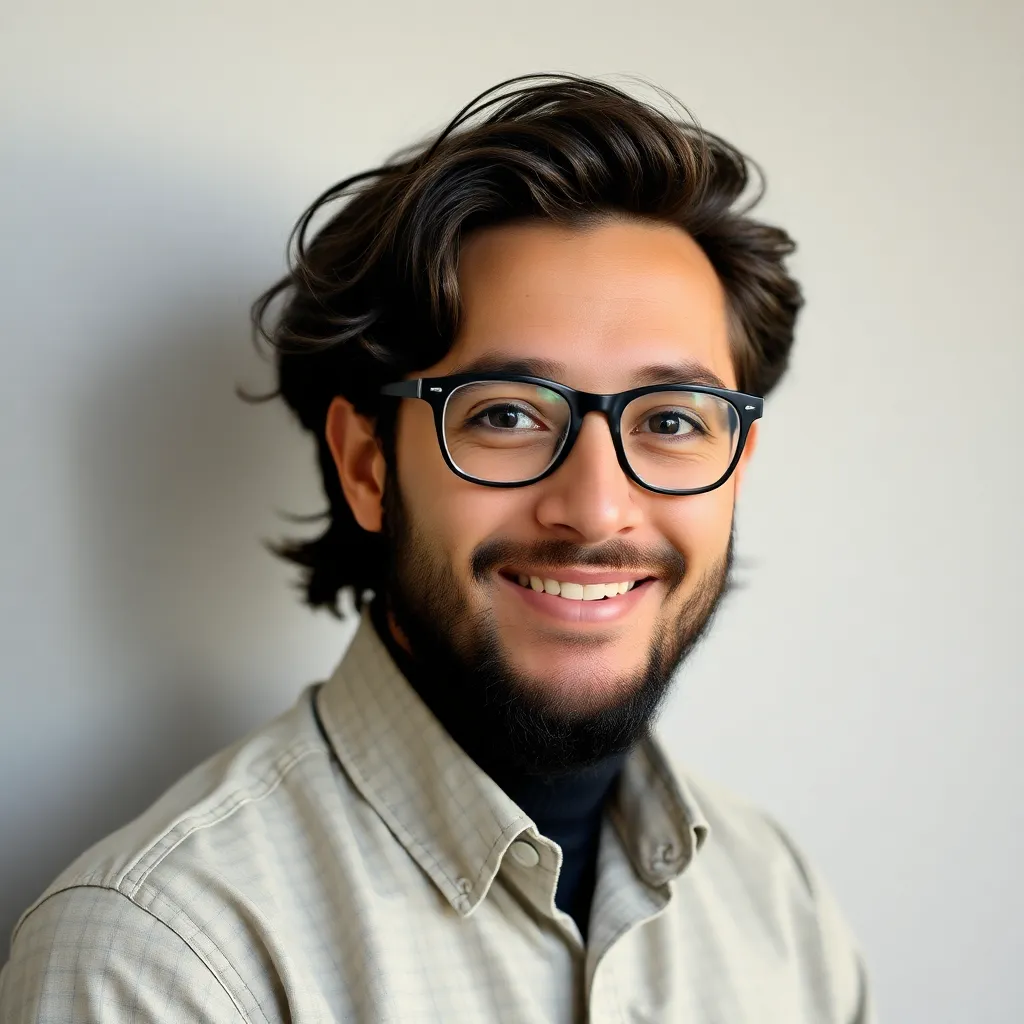
Muz Play
Apr 01, 2025 · 5 min read
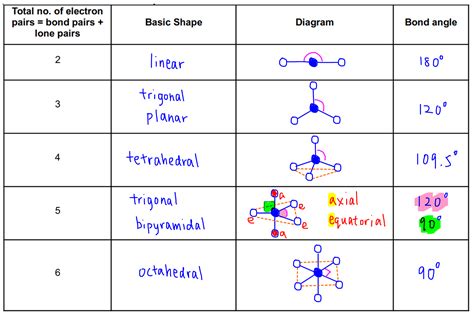
Table of Contents
What is the VSEPR Geometry of a Particle? A Comprehensive Guide
Understanding the shape of molecules is crucial in chemistry. It dictates their properties, reactivity, and interactions with other molecules. The Valence Shell Electron Pair Repulsion (VSEPR) theory provides a simple yet powerful model for predicting molecular geometry. This comprehensive guide delves into the intricacies of VSEPR theory, explaining its principles, applications, and limitations. We will explore various molecular geometries, providing examples and illustrating how to determine the shape of a molecule using this theory.
Understanding VSEPR Theory: The Basics
At its core, VSEPR theory posits that the electron pairs surrounding a central atom in a molecule will arrange themselves to minimize electrostatic repulsion. This minimization leads to specific three-dimensional arrangements, defining the molecule's geometry. The theory considers both bonding pairs (electrons shared between atoms) and lone pairs (electrons not involved in bonding) in determining the overall shape.
Key Principles:
- Electron Pair Repulsion: The fundamental principle is that electron pairs, whether bonding or lone, repel each other. They try to get as far apart as possible.
- Lone Pair vs. Bonding Pair Repulsion: Lone pairs occupy more space than bonding pairs. This is because lone pairs are attracted to only one nucleus, while bonding pairs are attracted to two. Consequently, lone pairs exert a stronger repulsive force, influencing the molecular geometry.
- Steric Number: The steric number is the sum of the number of bonding pairs and lone pairs around the central atom. This number is crucial in predicting the electron-pair geometry.
- Molecular Geometry vs. Electron-Pair Geometry: Electron-pair geometry describes the arrangement of all electron pairs (bonding and lone) around the central atom. Molecular geometry, however, only considers the arrangement of bonding pairs, as this dictates the actual shape of the molecule.
Predicting Molecular Geometry: A Step-by-Step Approach
Predicting the VSEPR geometry involves a systematic approach:
-
Draw the Lewis Structure: The first step is to accurately draw the Lewis structure of the molecule. This shows the arrangement of atoms and valence electrons.
-
Determine the Steric Number: Count the number of bonding pairs and lone pairs around the central atom. The sum is the steric number.
-
Identify the Electron-Pair Geometry: Based on the steric number, determine the electron-pair geometry. Common electron-pair geometries include linear (steric number 2), trigonal planar (3), tetrahedral (4), trigonal bipyramidal (5), and octahedral (6).
-
Determine the Molecular Geometry: This step considers the effect of lone pairs. Lone pairs influence the bond angles and the overall shape of the molecule. The presence of lone pairs distorts the ideal geometry predicted by the electron-pair geometry.
-
Name the Molecular Geometry: Based on the arrangement of bonding pairs, assign the appropriate molecular geometry name.
Common Molecular Geometries and Examples
Let's examine some common molecular geometries and illustrate them with examples:
1. Linear (Steric Number 2):
- Electron-pair geometry: Linear
- Molecular geometry: Linear
- Example: BeCl₂ (Beryllium chloride) - Two bonding pairs, no lone pairs. The chlorine atoms are 180° apart.
2. Trigonal Planar (Steric Number 3):
- Electron-pair geometry: Trigonal planar
- Molecular geometry: Trigonal planar (no lone pairs), Bent (one lone pair)
- Examples:
- BF₃ (Boron trifluoride) - Three bonding pairs, no lone pairs. The fluorine atoms are 120° apart.
- SO₂ (Sulfur dioxide) - Two bonding pairs, one lone pair. The oxygen atoms are less than 120° apart due to lone pair repulsion.
3. Tetrahedral (Steric Number 4):
- Electron-pair geometry: Tetrahedral
- Molecular geometry: Tetrahedral (no lone pairs), Trigonal pyramidal (one lone pair), Bent (two lone pairs)
- Examples:
- CH₄ (Methane) - Four bonding pairs, no lone pairs. The hydrogen atoms are approximately 109.5° apart.
- NH₃ (Ammonia) - Three bonding pairs, one lone pair. The hydrogen atoms are approximately 107° apart.
- H₂O (Water) - Two bonding pairs, two lone pairs. The hydrogen atoms are approximately 104.5° apart.
4. Trigonal Bipyramidal (Steric Number 5):
- Electron-pair geometry: Trigonal bipyramidal
- Molecular geometries: Trigonal bipyramidal (no lone pairs), Seesaw (one lone pair), T-shaped (two lone pairs), Linear (three lone pairs)
- Example: PCl₅ (Phosphorus pentachloride) - Five bonding pairs, no lone pairs.
5. Octahedral (Steric Number 6):
- Electron-pair geometry: Octahedral
- Molecular geometries: Octahedral (no lone pairs), Square pyramidal (one lone pair), Square planar (two lone pairs)
- Example: SF₆ (Sulfur hexafluoride) - Six bonding pairs, no lone pairs.
Beyond the Basics: Exceptions and Limitations
While VSEPR theory is remarkably successful in predicting molecular geometries, it has limitations:
- Multiple Central Atoms: VSEPR struggles with molecules containing multiple central atoms, where interactions between these atoms become significant.
- Transition Metal Complexes: The theory is less accurate for transition metal complexes due to the involvement of d-orbitals and more complex bonding scenarios.
- Highly Symmetrical Molecules: Some highly symmetrical molecules exhibit deviations from ideal bond angles predicted by VSEPR.
- Electron Delocalization: In molecules with resonance structures, the actual geometry can deviate from predictions based on a single Lewis structure.
Advanced Applications and Considerations
VSEPR theory forms the foundation for understanding many chemical concepts:
- Polarity: Molecular geometry influences the polarity of a molecule, which affects its physical and chemical properties. Asymmetrical geometries often result in polar molecules.
- Spectroscopy: The predicted geometry helps interpret spectral data, such as infrared and Raman spectroscopy, by correlating vibrational modes with the molecular shape.
- Reactivity: The accessibility of different regions of a molecule, governed by its geometry, greatly affects its reactivity.
Conclusion: A Powerful Tool in Molecular Structure Prediction
VSEPR theory offers a relatively simple yet effective method for predicting molecular geometry. Understanding its principles, applying the systematic approach, and recognizing its limitations empower chemists to interpret molecular structures and predict properties. While advanced techniques might be needed for complex molecules, VSEPR provides a crucial foundational understanding of molecular shapes, paving the way for more intricate analyses. Its widespread application in various chemical disciplines solidifies its status as an indispensable tool in the chemist's arsenal. The ability to visualize and understand 3D molecular structures is fundamental to many areas of chemistry, and VSEPR theory provides the essential framework for doing so. Remember to always consider the nuances and exceptions, ensuring a comprehensive understanding of molecular geometry.
Latest Posts
Latest Posts
-
What Does Rolling Without Slipping Mean
Apr 02, 2025
-
The Price Elasticity Of Supply Measures
Apr 02, 2025
-
Does Co Have Dipole Dipole Forces
Apr 02, 2025
-
What Are The Characteristics Of Enzymes
Apr 02, 2025
-
What Is The Tension In The Rope
Apr 02, 2025
Related Post
Thank you for visiting our website which covers about What Is The Vsepr Geometry Of The Particle . We hope the information provided has been useful to you. Feel free to contact us if you have any questions or need further assistance. See you next time and don't miss to bookmark.