Animal Cell In Hypertonic Solution What Happens
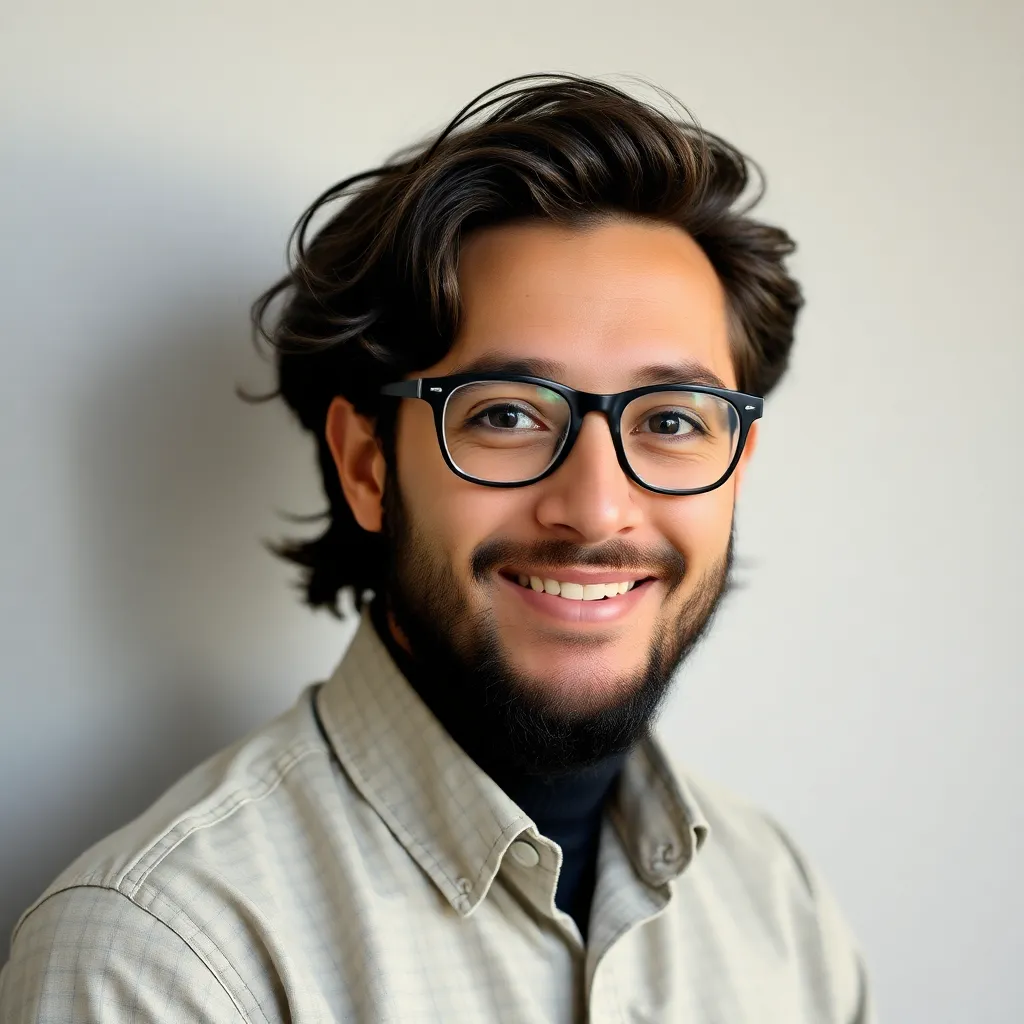
Muz Play
Mar 30, 2025 · 7 min read
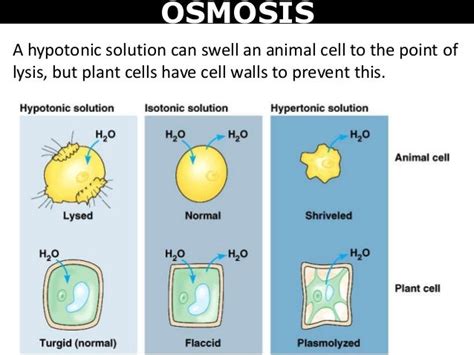
Table of Contents
Animal Cell in a Hypertonic Solution: What Happens? A Comprehensive Guide
Understanding the behavior of animal cells in different environments is crucial in biology. One key concept is the impact of tonicity, specifically hypertonic solutions, on animal cell structure and function. This article delves deep into the effects of a hypertonic solution on an animal cell, exploring the underlying mechanisms and consequences. We'll cover the basics of osmosis and tonicity, examine the cellular response to hypertonic stress, and discuss the implications for various biological processes.
Understanding Osmosis and Tonicity
Before diving into the specifics of hypertonic solutions and their impact on animal cells, let's establish a solid foundation in osmosis and tonicity.
Osmosis: The Movement of Water
Osmosis is the passive movement of water molecules across a selectively permeable membrane from a region of higher water concentration to a region of lower water concentration. This movement continues until equilibrium is reached, meaning the water concentration is equal on both sides of the membrane. The driving force behind osmosis is the difference in water potential between the two regions. Water always moves towards an area with a lower water potential, which is often associated with a higher solute concentration.
Tonicity: Comparing Solute Concentrations
Tonicity refers to the relative concentration of solutes dissolved in a solution compared to the concentration of solutes inside a cell. This comparison is crucial for determining the direction of water movement across the cell membrane. There are three main types of tonicity:
- Isotonic: The solution has the same solute concentration as the inside of the cell. There is no net movement of water; water moves in and out at equal rates.
- Hypotonic: The solution has a lower solute concentration than the inside of the cell. Water moves into the cell, causing it to swell and potentially burst (lyse).
- Hypertonic: The solution has a higher solute concentration than the inside of the cell. Water moves out of the cell, causing it to shrink and shrivel (crenate). This is the focus of this article.
Animal Cell in a Hypertonic Solution: The Process of Crenation
When an animal cell is placed in a hypertonic solution, the concentration of solutes outside the cell is higher than inside. This creates a water potential gradient, driving water out of the cell across the selectively permeable cell membrane via osmosis. The result is crenation, a process where the cell shrinks and its membrane pulls away from the cell wall (if present, as in plant cells; animal cells lack cell walls).
Step-by-Step Breakdown of Crenation:
-
Water Potential Gradient: The initial difference in water potential between the hypertonic solution and the cell's cytoplasm triggers the movement of water.
-
Water Efflux: Water molecules move across the cell membrane from the area of higher water potential (inside the cell) to the area of lower water potential (the hypertonic solution). This is a passive process, requiring no energy input from the cell.
-
Cell Shrinkage: As water leaves the cell, the cell's cytoplasm decreases in volume. The cell membrane, being flexible, pulls away from the cell's contents, leading to the characteristic shrunken appearance.
-
Cytoplasmic Concentration Changes: The concentration of solutes within the cytoplasm increases as the water volume decreases. This change can impact various cellular processes.
-
Cellular Dysfunction: The extreme shrinkage associated with crenation can disrupt the cell's normal function. Organelles can be compressed, and essential cellular processes may be impaired.
Consequences of Crenation: Cellular Impacts and Biological Implications
The effects of crenation on an animal cell extend beyond simple shrinkage. The process can significantly alter cellular structure and function, leading to a range of consequences:
1. Membrane Damage:
Severe crenation can cause damage to the cell membrane. The stress exerted on the membrane as it shrinks can lead to structural disruption, potentially causing tears or compromising its integrity. This compromises the cell's ability to regulate the passage of substances and maintain homeostasis.
2. Organelle Dysfunction:
The reduced cell volume and altered cytoplasmic concentration can affect the function of various organelles. For instance, the endoplasmic reticulum, responsible for protein synthesis and lipid metabolism, can be compressed, impacting its efficiency. Mitochondria, the powerhouses of the cell, might also be affected, leading to reduced ATP production and impaired cellular energy levels.
3. Impaired Metabolic Processes:
Crenation disrupts the normal transport of substrates and products involved in metabolic reactions. Enzyme activity can be affected by the altered cytoplasmic concentration. The decreased cell volume limits the available space for metabolic processes, leading to impaired cellular function and potentially cell death.
4. Cellular Signaling Disruption:
The cell membrane plays a critical role in cell signaling, acting as a receptor for various external signals. Crenation alters the cell membrane structure and can impair its ability to receive and respond to these signals properly. This disruption can have significant consequences for cell communication and coordination within tissues and organs.
5. Cell Death (Necrosis):
In extreme cases, prolonged exposure to a hypertonic solution can lead to irreversible cell damage and death through necrosis. Necrosis is a form of cell death characterized by swelling and rupture of the cell membrane, resulting in the release of cellular contents into the surrounding environment. This can trigger inflammation and damage to surrounding tissues.
Examples of Hypertonic Environments in Biology
Hypertonic environments are not just laboratory constructs; they occur naturally in various biological contexts. Understanding these contexts helps appreciate the importance of osmoregulation, the process by which cells maintain their internal water balance.
1. Marine Environments:
Many marine organisms live in environments with high salt concentrations. Their cells are constantly exposed to the risk of crenation due to the hypertonic nature of seawater. These organisms have evolved sophisticated mechanisms to regulate their internal osmolarity, preventing excessive water loss and maintaining cell integrity.
2. Dehydration:
In animals, dehydration can lead to a hypertonic internal environment. As water is lost from the body, the concentration of solutes in the blood and other bodily fluids increases. This can cause cells to crenate, potentially leading to organ dysfunction and various health problems.
3. Food Preservation:
Hypertonic solutions are used in food preservation techniques like pickling and salting. The high salt concentration creates a hypertonic environment that inhibits microbial growth by causing water loss from microbial cells, thus preventing spoilage.
Cellular Responses to Hypertonic Stress
Animal cells have evolved various strategies to cope with hypertonic stress and minimize the effects of crenation. These responses aim to maintain cell integrity and function despite the water loss.
1. Osmolyte Accumulation:
Cells can accumulate compatible organic solutes, known as osmolytes, within their cytoplasm. These osmolytes help balance the osmotic pressure difference between the cell and its surroundings without interfering with cellular processes. Examples include amino acids, polyols, and methylamines.
2. Ion Channel Regulation:
Cells can regulate the transport of ions across their membranes to adjust the osmotic pressure. This might involve increasing the influx of certain ions or decreasing the efflux of others. Careful control over ion channels helps mitigate water loss.
3. Aquaporin Regulation:
Aquaporins are water channels in the cell membrane that facilitate water movement. Cells can modulate the expression or activity of aquaporins to control the rate of water transport in response to osmotic stress.
4. Cellular Signaling Pathways:
Hypertonic stress triggers various cellular signaling pathways that activate protective mechanisms. These pathways involve various signaling molecules, including kinases and transcription factors, that coordinate the cellular response to osmotic stress.
Conclusion: The Significance of Understanding Hypertonic Environments
Understanding the effects of hypertonic solutions on animal cells is fundamental to various biological disciplines. From understanding the adaptation of marine organisms to developing new medical treatments, the impact of osmosis and tonicity is far-reaching. The information presented here serves as a foundation for more advanced explorations of cellular physiology, environmental adaptations, and the development of therapeutic strategies to address osmotic stress-related diseases. Further research continues to reveal the intricacies of cellular responses to hypertonic environments, constantly improving our understanding of this vital aspect of cell biology. This knowledge can be applied to diverse fields, such as agriculture, medicine, and environmental science, paving the way for advancements in these areas.
Latest Posts
Latest Posts
-
Moment Of Inertia Of A Point
Apr 01, 2025
-
Cross Section Of A Woody Stem
Apr 01, 2025
-
A State Function Is Best Described As
Apr 01, 2025
-
What Is The Electron Configuration For Ne
Apr 01, 2025
-
Difference Between E1 And E2 Reaction
Apr 01, 2025
Related Post
Thank you for visiting our website which covers about Animal Cell In Hypertonic Solution What Happens . We hope the information provided has been useful to you. Feel free to contact us if you have any questions or need further assistance. See you next time and don't miss to bookmark.