The Three Main Biochemical Pathways Of Cellular Respiration Are
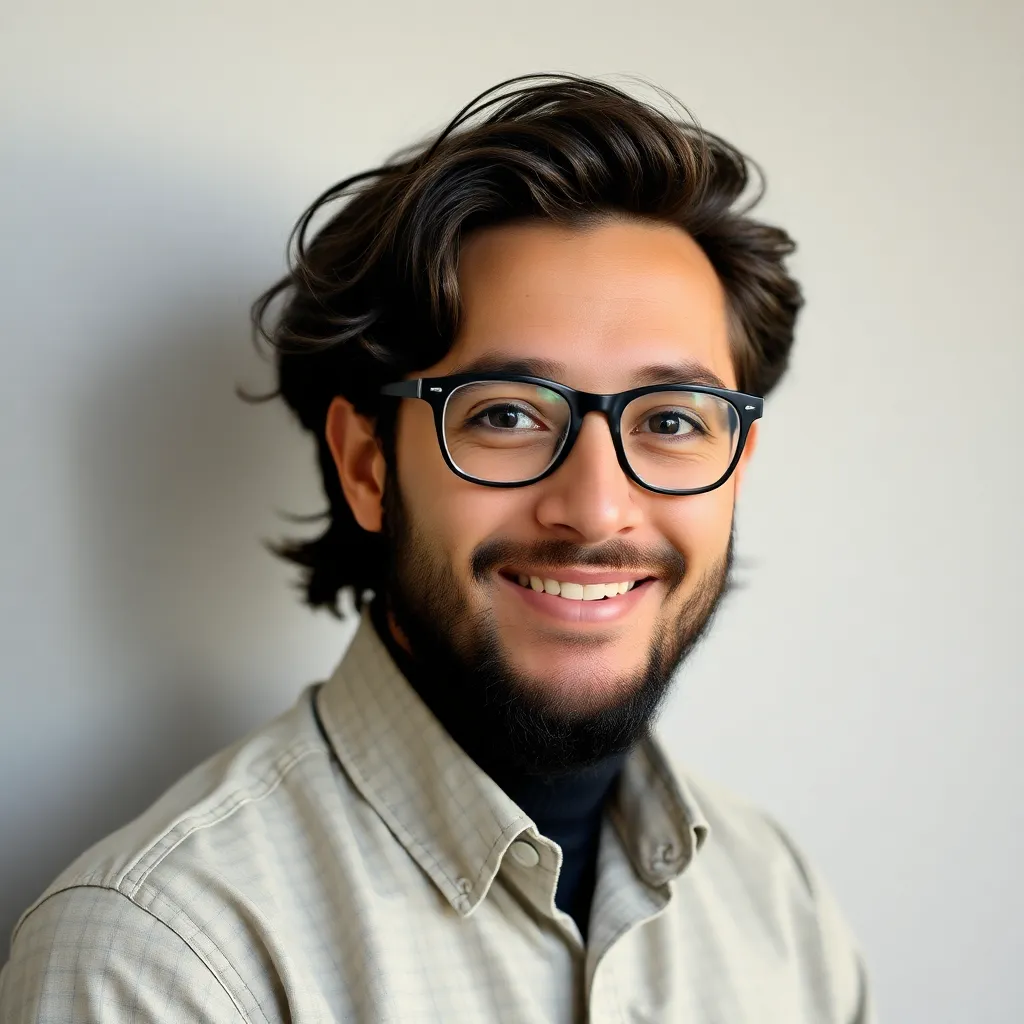
Muz Play
Mar 30, 2025 · 7 min read
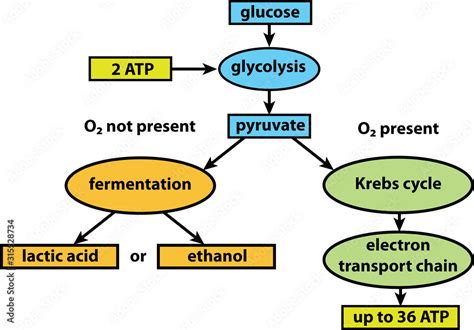
Table of Contents
The Three Main Biochemical Pathways of Cellular Respiration: A Deep Dive
Cellular respiration is the fundamental process by which living cells break down organic molecules, primarily glucose, to generate energy in the form of ATP (adenosine triphosphate). This energy fuels a vast array of cellular activities, from muscle contraction and protein synthesis to nerve impulse transmission and maintaining cellular homeostasis. This intricate process isn't a single event but a series of interconnected biochemical pathways, each with its unique role and location within the cell. This article delves into the three main pathways: glycolysis, the citric acid cycle (also known as the Krebs cycle or tricarboxylic acid cycle), and oxidative phosphorylation (including the electron transport chain and chemiosmosis).
1. Glycolysis: The Initial Breakdown of Glucose
Glycolysis, meaning "sugar splitting," is the first stage of cellular respiration and the only one that occurs in the cytoplasm, outside the mitochondria. It's an anaerobic process, meaning it doesn't require oxygen. This ten-step pathway breaks down a single molecule of glucose (a six-carbon sugar) into two molecules of pyruvate (a three-carbon compound). This breakdown releases a small amount of energy, captured in the form of ATP and NADH (nicotinamide adenine dinucleotide), a crucial electron carrier.
Key Steps and Energy Production in Glycolysis:
Glycolysis can be broadly divided into two phases: the energy investment phase and the energy payoff phase.
-
Energy Investment Phase: This initial phase consumes two ATP molecules to phosphorylate glucose and its subsequent intermediates. This phosphorylation primes the glucose molecule for the subsequent breakdown. The key enzyme here is hexokinase.
-
Energy Payoff Phase: This phase yields four ATP molecules and two NADH molecules per glucose molecule. The key enzymes in this phase include glyceraldehyde-3-phosphate dehydrogenase and pyruvate kinase.
Net Gain in Glycolysis: While four ATP molecules are produced, two are consumed in the initial phase, resulting in a net gain of two ATP molecules and two NADH molecules per glucose molecule. This relatively small energy yield sets the stage for the significantly larger energy harvest in the subsequent stages.
Regulation of Glycolysis: The rate of glycolysis is tightly regulated to meet the cell's energy demands. Key regulatory enzymes, such as hexokinase, phosphofructokinase, and pyruvate kinase, are subject to allosteric regulation by molecules like ATP, ADP, and citrate. High levels of ATP inhibit glycolysis, while high levels of ADP stimulate it, ensuring that ATP production is balanced with cellular energy needs.
Fate of Pyruvate: The pyruvate produced at the end of glycolysis has two main fates depending on the presence or absence of oxygen:
-
Aerobic Conditions (Presence of Oxygen): Pyruvate enters the mitochondria and is converted into acetyl-CoA, entering the citric acid cycle.
-
Anaerobic Conditions (Absence of Oxygen): Pyruvate undergoes fermentation, either lactic acid fermentation (in animals and some bacteria) or alcoholic fermentation (in yeast and some bacteria). This process regenerates NAD+ from NADH, allowing glycolysis to continue despite the lack of oxygen. However, it yields significantly less ATP than aerobic respiration.
2. Citric Acid Cycle (Krebs Cycle or TCA Cycle): Central Hub of Metabolism
The citric acid cycle, also known as the Krebs cycle or tricarboxylic acid (TCA) cycle, takes place within the mitochondrial matrix. It's an aerobic process, requiring oxygen indirectly, as oxygen is the ultimate electron acceptor in the electron transport chain. Here, the acetyl-CoA produced from pyruvate is completely oxidized, releasing carbon dioxide as a byproduct and generating high-energy electron carriers (NADH and FADH2 – flavin adenine dinucleotide).
Detailed Steps of the Citric Acid Cycle:
The citric acid cycle is a cyclical pathway, with each turn processing one acetyl-CoA molecule. The key steps involve a series of enzyme-catalyzed reactions:
-
Acetyl-CoA + Oxaloacetate → Citrate: The cycle begins with the condensation of acetyl-CoA (two carbons) and oxaloacetate (four carbons) to form citrate (six carbons).
-
Citrate Isomerized to Isocitrate: Citrate undergoes isomerization to form isocitrate.
-
Isocitrate → α-Ketoglutarate: Isocitrate is oxidized and decarboxylated (loses a CO2 molecule) to form α-ketoglutarate. This step produces one NADH molecule.
-
α-Ketoglutarate → Succinyl-CoA: α-Ketoglutarate is further oxidized and decarboxylated to form succinyl-CoA. This step produces another NADH molecule.
-
Succinyl-CoA → Succinate: Succinyl-CoA is converted to succinate, generating one GTP (guanosine triphosphate) molecule, which is readily converted to ATP.
-
Succinate → Fumarate: Succinate is oxidized to fumarate, producing one FADH2 molecule.
-
Fumarate → Malate: Fumarate is hydrated to form malate.
-
Malate → Oxaloacetate: Malate is oxidized to regenerate oxaloacetate, producing one more NADH molecule.
Net Gain per Acetyl-CoA in the Citric Acid Cycle: For each acetyl-CoA molecule entering the cycle, the net gain is:
- 3 NADH molecules
- 1 FADH2 molecule
- 1 ATP (or GTP) molecule
- 2 CO2 molecules
Since each glucose molecule produces two pyruvate molecules (and thus two acetyl-CoA molecules), the total yield from one glucose molecule is doubled.
Regulation of the Citric Acid Cycle: Similar to glycolysis, the citric acid cycle is tightly regulated to maintain energy balance. Key regulatory enzymes, such as citrate synthase, isocitrate dehydrogenase, and α-ketoglutarate dehydrogenase, are sensitive to the energy charge of the cell (ATP/ADP ratio) and the levels of other metabolites. High levels of ATP or NADH inhibit the cycle, while low levels stimulate it.
3. Oxidative Phosphorylation: The Powerhouse of Cellular Respiration
Oxidative phosphorylation, the final stage of cellular respiration, occurs in the inner mitochondrial membrane. It involves two closely coupled processes: the electron transport chain (ETC) and chemiosmosis. This stage generates the vast majority of ATP produced during cellular respiration.
The Electron Transport Chain (ETC): A Cascade of Redox Reactions
The electron transport chain consists of a series of protein complexes embedded in the inner mitochondrial membrane. Electrons from NADH and FADH2, generated in glycolysis and the citric acid cycle, are passed along this chain through a series of redox reactions (reduction-oxidation). As electrons move down the chain, their energy is progressively released, used to pump protons (H+) from the mitochondrial matrix into the intermembrane space.
Components of the ETC: The ETC consists of four major protein complexes (I-IV), along with two mobile electron carriers, ubiquinone (CoQ) and cytochrome c.
- Complex I (NADH dehydrogenase): Receives electrons from NADH.
- Complex II (succinate dehydrogenase): Receives electrons from FADH2.
- Complex III (cytochrome bc1 complex): Transfers electrons from ubiquinone to cytochrome c.
- Complex IV (cytochrome c oxidase): Transfers electrons to molecular oxygen (O2), the final electron acceptor, forming water.
Chemiosmosis: Harnessing the Proton Gradient
The pumping of protons into the intermembrane space by the ETC creates a proton gradient, also known as a proton motive force. This gradient stores potential energy. This energy is then harnessed by ATP synthase, an enzyme embedded in the inner mitochondrial membrane.
ATP Synthase and ATP Production: ATP synthase utilizes the energy from the proton gradient to synthesize ATP from ADP and inorganic phosphate (Pi). Protons flow down their concentration gradient, through ATP synthase, driving the rotation of a part of the enzyme, which in turn catalyzes the synthesis of ATP. This process is called chemiosmosis.
ATP Yield in Oxidative Phosphorylation: The exact ATP yield varies slightly depending on the efficiency of the electron transport chain and the shuttle systems used to transport NADH from the cytoplasm to the mitochondria. However, a commonly accepted estimate is approximately 32 ATP molecules per glucose molecule, the majority coming from oxidative phosphorylation.
Overall ATP Yield from Cellular Respiration:
The total ATP yield from the complete oxidation of one glucose molecule through cellular respiration is approximately 36-38 ATP molecules. This number is a summation of the ATP produced in glycolysis (2 ATP), the citric acid cycle (2 ATP), and oxidative phosphorylation (32-34 ATP).
Conclusion: A Highly Regulated and Efficient Process
Cellular respiration is a remarkably efficient and tightly regulated process that extracts a substantial amount of energy from glucose. The three main biochemical pathways—glycolysis, the citric acid cycle, and oxidative phosphorylation—work in concert to generate ATP, the primary energy currency of the cell. Understanding these pathways is crucial for comprehending numerous biological processes and developing strategies to combat various diseases associated with metabolic dysfunction. Further research continues to unveil the intricate details of this fundamental biological process, opening up new avenues for therapeutic interventions.
Latest Posts
Latest Posts
-
Identify The Body Cavities In The Following Illustration
Apr 01, 2025
-
How Did Louis Pasteur Disprove Spontaneous Generation
Apr 01, 2025
-
Which Electrons Are Involved In Chemical Bonding
Apr 01, 2025
-
How Do You Calculate Stream Gradient
Apr 01, 2025
-
What Happens To A Cell In A Hypertonic Solution
Apr 01, 2025
Related Post
Thank you for visiting our website which covers about The Three Main Biochemical Pathways Of Cellular Respiration Are . We hope the information provided has been useful to you. Feel free to contact us if you have any questions or need further assistance. See you next time and don't miss to bookmark.